INTRODUCTION
Polylepis is a genus that includes 28 species of trees and shrubs distributed throughout the Andes Mountains, from the north of Venezuela to the north of Chile and Argentina, mainly between 3,500-4,800 m.a.s.l.1,2The genus is characterized by having compound leaves and a woody trunk that has outer layers, called rhytidome, dead bark tissue that is shed throughout the life cycle of the species. In Peru it is known as Queñual and 19 species are reported, being in this way the country that presents the greatest diversity of this genus.3 It is used to extract a natural dye, in traditional medicine, as fuel and as food for animals.4
There are not many studies of the secondary metabolites present in Polylepis species. The ethanolic extract of Polylepis tomentella leaves is known to contain oleanolic acid, 4.49 mg/g, determined by HPLC-DAD.5 On the other hand, from a CHCl3-MeOH extract from P. incana leaves it was possible to isolate and identify triterpenoids (nigaichigoside, 23-hydroxytormentic acid, corosolic acid) and flavonoids (kaempferol, quercetin, catechin, quercetin-3-galactoside, quercetin-3-glucoside, kaempferol-3-O-β-D- (6'-O-feruloylglucopyranoside, tiliroside, quercetin-3-arabinoside, apigenin and luteolin).6,7
On the other hand, silver nanoparticles (AgNPs) are being intensively studied due to their multiple applications (catalysis, antibacterial, biosensors, and surface-enhanced Raman scattering (SERS)).8 Ag nanoparticle is an interesting material, especially in the field of medicine. The AgNPs are strong antibacterial due to their ability to damage bacterial cell walls (decreases the membrane permeability), inhibit bacterial cell growth, and disrupt cell metabolism because of the interaction between Ag ions with macromolecules (proteins, DNA, etc.) in cells, and eventually leads to cell death.9 The properties of AgNPs largely depend on the shape and size of the nanoparticles. In general, for the synthesis of AgNPs a precursor (AgNO3), a reducing agent (to carry out the Ag+ → Ag0 process) and a stabilizing agent of AgNPs (to control their size) are required. Depending on the type of reducing agent used, the synthesis of AgNPs can be classified as chemical, biological and physical.10 Each of these methods has its advantages and disadvantages.
The chemical method is by far the most widely used to prepare AgNPs. However, the use of chemicals in the synthesis of AgNPs results in the adsorption of toxic chemicals (reducing agents, stabilizing agents and organic solvents) on the surface of the material so that it will have adverse and harmful effects, especially on biomedical applications. Green synthesis methods for synthesizing nanoparticles using natural products can be used to address the problem by utilizing plant extract (source of reducing secondary metabolites) or microorganisms (such as yeast, fungi, and bacteria) which provide reducing and stabilizing agents.9,11 Biomolecules present in plant extracts can be used to reduce metal ions to nanoparticles in a single-step green synthesis process. Additionally, the biosynthesized AgNPs exhibit good biological activity with less cytotoxicity.12 This biogenic reduction of metal ions to base metal is quite rapid, readily conducted at room temperature and pressure, and easily scaled up. The involved reducing agents include the various water-soluble plant metabolites (e.g. alkaloids, phenolic compounds, terpenoids) and co-enzymes. AgNPs have a particular focus on plant-based synthesis.13 Within this category, most of the works use extracts from leaf,14 or bark,15,16 but there are other biological materials used to obtain AgNPs.17
This work presents a study on the secondary metabolites present in an aqueous extract of Polylepis racemosa rhytidomes carried out by UHPLC-ESI-MS/MS. It discusses the influence of the composition of the extracts on the green synthesis of silver nanoparticles.
EXPERIMENTAL SECTION
Material
Polylepis racemosa specimen was collected at Chinancocha lac (9°04'44.4"S 77°39'03.2"W) at Yungay, Ancash, Peru, in 2017 at an altitude of 3850 m. A voucher specimen (register 316186) was deposited in the Museo de Historia Natural from the Universidad Mayor de San Marcos (Lima, Peru) and biologists Mg. A. Cano and W. Mendoza confirmed its identity.
Rhytidome extracts
The clean and mechanically purified rhytidome was carefully separated into its sheets, cut, weighed and covered with filter paper, then immersed in ultra-pure water (Type I), previously filtered (Millipore Type GNWP 0.20 µm). The extraction (0.9 grams of rhytidomes per 35 mL of water) was carried out at room temperature and protected from sunlight, during the established time of 2, 6, 12 and 30 minutes for each extract, called Extract 2, Extract 6, Extract 12 and Extract 30, correspondingly.
Synthesis of AgNPs
5 mL of extract (0.9 g of rhytidomes/35 mL of H2O) and 1 mL of 0.01 M AgNO3 solution were mixed with minimal stirring, at room temperature, protected from light and kept refrigerated. For their subsequent characterization, the AgNPs solutions were centrifuged at 6000 RPM for 30 minutes (Ultracentrifuge LABWE model BT20R). The UV-Vis, DLS, UHPLC-ESI-MS / MS readings were performed after 14 hours of synthesis.
Characterization
pH and conductivity.- Aqueous extracts of Polylepis racemosa (0.9 g of rhytidomes/35 mL of H2O) were stirred at 400 rpm and pH and conductivity readings were made every 30 seconds with a Milwaukee MW 801 conductivity meter calibrated with standards, at 27.3 ºC.
UV-Vis.- Aqueous extracts of Polylepis racemosa (0.9 g of rhytidomes/35 mL of H2O) with different extraction times, and colloidal solutions of AgNPs, were read with a UV-Vis Thermo Scientific Model Hελios γ spectrophotometer, with quartz cells, between 200 and 600 nm, with water as blank.
Voltammetry.- 1 mL of an aqueous extract of P. racemosa (1.0 g of rhytidomes/100 mL of H2O, 12 minutes of extraction) was diluted to 25 mL, with a phosphate buffer solution (pH 7 approximately). Working electrode: 3 mm diameter vitreous carbon, previously polished in 0.5-micron alumina, washed in deionized water and ultrasound. Counter electrode: Platinum wire. Reference Electrode: 1 M silver/silver chloride (Ag/AgCl) electrode. Nitrogen gas purging was performed for approximately 15 minutes before each measurement. Measurements were made with an AUTOLAB potentiostat.
Cyclic Voltammetry: From -0.2 V to 0.6 V, at a sweep speed of 100 mV / s and a potential step of 0.00244 mV. The voltamperogram of the blank, which contained 25 mL of phosphate buffer, was also determined.
Differential Pulse Voltammetry: The applied conditions were: Start potential: -0.5 V, End potential: 0.6 V, Step potential 0.005 V, Modulation amplitude: 0.025 V, Modulation time: 0.05 s, time interval 0.5 s.
DLS.- About 0.2 mL of the colloidal AgNPs were placed inside a Nicomp 380 nanoparticle size analyzer (Particle sizing system) to measure the hydrodynamic sizes of the AgNPs through programmed cycles of time and the results of the Dispersion are recorded using histograms of intensity, volume and number of particles
UHPLC-MS/MS.- Each of the samples (aqueous extracts or AgNPs colloidal solution) was filtered through a 0.45 µm syringe filter into a vial and the solution was stored at -20 °C until use. A volume of 10 µL was injected into the Dionex Ultimate 3000 UHPLC system (Thermo Scientific) Luna © Omega C18 100 Å Column, Phenomenex (150 mm x 2.1 mm x 1.6 µm). Column temperature: 30 °C. UV: 270, 280, 430 and 530 nm Flow: 0.3 mL/min Injection temperature: 18 °C Eluents: A: H2O 1% HCOOH (90%) B: Acetonitrile 1% HCOOH (10%). Mass spectrometer Instrument: Q Exactive Plus (Thermo Scientific) Full MS scan parameters Range: 110-1500 m/z Resolution: 35000 MS2 parameters Resolution: 17500 Ionization source parameters Ionization source and mode: ESI (positive and negative) Spray voltage: 3.5/2.5 KV Capillary temperature: 260 °C Carrier gas: N2 (Sheath gas flow rate: 48, Sweep gas flow rate: 2) Gas heater temp: 270/270 °C S-lens RF level: 100.
RESULTS AND DISCUSSION
Physicochemical characteristics of the extracts
pH and conductivity
The pH and conductivity data of the aqueous extracts of P. racemosa rhytidomes are shown in Figure 1. It is observed that as the extraction time increases, both the pH and the conductivity increase. The initial pH of the aqueous extract is slightly acidic, due to the presence of weak organic acids present. Then the pH is increased from 6.5 to 8.5. The same happens with the conductivity, varying from 10 to 100 μS/cm-1 as a function of the extraction time, which could be attributed to the flow of ions due to the osmotic effect of the interior of the rhytidomes cells of Polylepis racemosa.
Absorption spectra
Figure 2 corresponds to the scans of the aqueous extracts of P. racemosa rhytidomes, with extraction times of 2, 6, 12 and 30 min. Initially, the absorbance increases rapidly, reaching a maximum after 6 minutes of extraction, stabilizing until 12 minutes, and then decreasing rapidly.
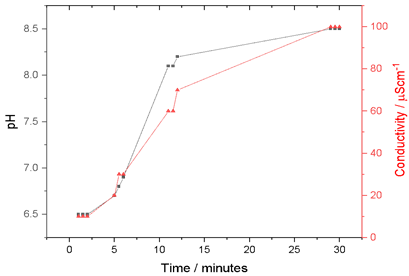
Figure 1 Evolution of pH and conductivity as a function of the extraction time of the rhytidomes in water.
Voltammetry
The cyclic voltammogram for the P. racemosa rhytidome sample for Extract 12 is shown in Figure 3a. The values of the anodic (Epa) and cathodic (Epc) peak potentials are detailed in the Table 1. The number of electrons transferred (n) was calculated by equation (1), while the half-wave potential was obtained by equation (2):
Table 1 Anodic and cathodic potentials of the aqueous extract of rhytidomes (12 minutes of extraction)
E p a (mV) | E p c (mV) | ΔE (Epa-Epc) | Electrons transferred (n) | Half wave potential E1/2 (mV) |
---|---|---|---|---|
242 | 168 | 74 | 0.79 | 205 |
The value of n, in this case, represents the average of many oxidation-reduction processes, so the value calculated for n is not very representative. Studies carried out on antioxidants found in plants show that reversible and irreversible transfer processes can occur, likewise more than one electron may be involved, due to the presence of more than one functional group as well as oxidative dimerization of these compounds.18,19 The differential pulse voltammogram shows a signal around 200 mV, ratifying what is observed by the cyclic voltammetry, Figure 3b. This potential is the one corresponding to the half-cell potential that can be calculated from the voltammetry cyclical. Additionally, a weak signal is also observed around -150 mV which can be attributed to some process that could not be observed by cyclic voltammetry. This weak signal could be related to the oxidation process of ellagic acid, which has been characterized as a pH-dependent, two-step quasi reversible process.20
Determination of the metabolites present in the aqueous extract of Polylepis racemosa rhytidomes
Thirteen compounds were identified for the first time in the aqueous extract from Polylepis racemosa. All of them were elucidated based on their ESI-MS-MS fragmentation patterns (negative mode) and UV data, and the use of a freely accessible database.21,22 Based on the examined information (Figure 4a and Table 2) it was identified uric acid, an amino acid derivative (N-acetylglutamic acid), three aliphatic carboxylic acids (malic acid, suberic acid and azelaic acid), two aromatic carboxylic acids (acid 3-, 4-dihydroxybenzoic acid, salicylic acid), a trihydroxylated carboxylic acid (9,12,13-trihydroxyoctadec-10-enoic acid), a polyphenol (Ellagic acid 4-O-xylopyranoside) and three glycosylated triterpenes (2,3,6, 19-Tetrahydroxy-12-oleanen-28-O-glucopyranosyl ester, rosamultin, 2,3,19-Trihydroxy-12-ursen-28-oic acid). These results are summarized in Table 1. All these compounds are new for P. racemosa, since it has not been studied much, however, compound 8 has a molecular peak and fragmentation that does not appear in the latest version of the Natural Products Dictionary database, so we assume it's a new metabolite and call it racemosaoic acid.
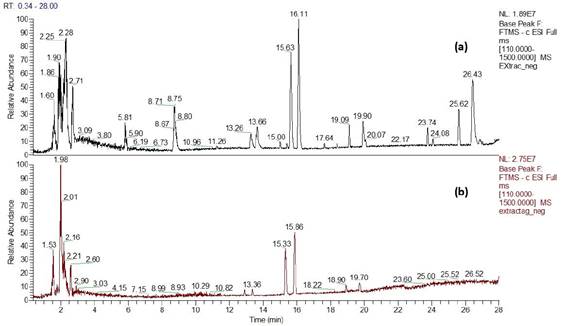
Figure 4 Chromatogram in the negative mode of the aqueous extract of P. racemosa rhytidome, obtained by UHPLC-MS. a) Avant and b) before AgNPs synthesis.
The carboxylic acids, compounds 2, 3, 4, 5, 6, 9 and 13, show the characteristic fragmentation (loss of the OH group, in the form of water, and of the carboxylic group). However, compound 12 did not present the loss of the carboxylic group in its fragmentation, but instead presented the loss of successive three water molecules. Compound 7, ellagic acid 4-O-xylopyranoside (tR = 13.26 min) presents, apart from the molecular peak (M-H) - at 433.0414 (C19H14O12; 434.0414), an m/Z signal, at 300.9990, corresponding to the loss of the xylopyranoside group.23
Table 2 Compounds identified by UHPLC-MS from the aqueous extract of Polylepis racemosa, with 12 minutes of extraction.
Compound | tR (min) | MS-ES- | Nominal MW | Molecular Formule | Chemical structure |
1 | 2.08 | 167.0204 [M-H] | 168 | C5H4N4O3 | Uric acid |
2 | 2.28 | 133.0134 [M-H] | 134 | C4H6O5 | Malic acid |
3 | 2.71 | 188.0560 [M-H] | 189 | C7H11NO5 | N-Acetyl-L-glutamic acid |
4 | 5.81 | 153.0187 [M-H] | 154 | C7H6O4 | 3,4-Dihydroxybenzoic acid |
5 | 8.75 | 137.0237 [M-H] | 138 | C7H6O3 | Salicylic acid |
6 | 13.26 | 173.0814 [M-H] | 174 | C8H14O4 | Suberic acid |
7 | 13.26 | 433.0414 [M-H] | 434 | C19H14O12 | Ellagic acid 4-O-xylopyranoside |
8 | 13.66 | 491.0470 [M-H] | 492 | C21H16O14 | Racemosaoic acid |
9 | 15.63 | 187.0981 [M-H] | 188 | C9H16O4 | Azelaic acid |
10 | 16.11 | 711.3969 [M+FA-H] | 666 | C36H58O11 | 2,3,6,19-Tetrahydroxy-12-oleanen-28-O-Glucopyranosyl ester |
11 | 19.09 | 695.4016 [M+FA-H] | 650 | C36H58O10 | Rosamultin |
12 | 19.90 | 329.2335 | 330 | C18H34O5 | 9,12,13-trihydroxyoctadec-10-enoic acid |
13 | 23.74 | 293.1761 | 294 | C17H26O4 | Unknow |
14 | 25.62 | 487.3430 | 488 | C30H48O5 | 2,3,19-trihydroxy-12-ursen-28-oic acid |
Compound 8 (tR = 13.26 min) could not be unequivocally identified. The proposed structure was made based on the mass spectrum, which is typical for an ellagic acid derivative, with a methoxy group and a carboxylated xylopyranoside group. The mass spectrum of 8 (racemosaoic acid) presents, apart from the molecular peak (M-H) - at 491.0470 (C21H16O14), an m/Z signal, at 315.0150, corresponding to the loss of the xylopyranoside group, followed by the loss of a group methyl.24 The tentative structure of the compound is shown in Figure 5, however, to attribute the correct position of the methyl group and the sugar in the molecule, a subsequent nuclear magnetic resonance study will be necessary.
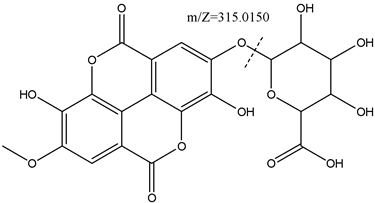
Figure 5 Racemosaoic acid (8), (6-((3,8-dihydroxy-7-methoxy-5,10-dioxo-5,10-dihydrochromeno[5,4,3-cde]chromen-2-yl)oxy)-3,4,5-trihydroxytetrahydro-2H-pyran-2-carboxylic acid), proposed structure, showing the loss of xylopyranoside group
Compounds 10 and 11 are structurally similar: they both have 5 fused rings linked by an ester to a glucopyranosyl group. The molecular peak is obtained by subtracting the molecular weight of formic acid, since the [M-FA-H] adduct was formed in both cases. One of the fragmentation peaks corresponds to the loss of the glucopyranosyl group, followed by the loss of H2O.25
It is interesting to note the characteristics of the aqueous extract of P. racemosa as a source of carboxylic acids (especially dicarboxylic acids), glycosylated compounds and saponins. The case of the glycosylated derivatives of ellagic acid is worth noting since it would be a solution to the low solubility and bioavailability of ellagic acid, which has been reported to exert a beneficial effect against severe pathologies associated with oxidative damage (cancer and diseases cardiovascular and neurodegenerative).26
Likewise, the anti-biofilm activity of glycosylated ellagic acid has been reported (Fontaine, 2017). Similarly, Rosamultin (11), a pentacyclic triterpenoid saponin, has pharmacological properties.27
Synthesis of silver nanoparticles
For this synthesis, extracts of P. racemosa were used with extraction times of 2, 6, 12 and 30 minutes. In all cases, the reaction mixture rapidly changed color to reddish brown and became slightly cloudy.
Absorption spectrum of AgNPs
The absorption spectrum of these mixtures, Figure 6, shows that the highest absorbance, in the UV range, corresponds to the 2-minute extract, and then decreases to 12 minutes. After this time there are no more changes: the 12 and 30-minute curves are identical. However, comparing Figures 3 and 6 it is observed that the extracts before the synthesis of AgNPs show well-differentiated bands one at 213.5 nm with 1.44 U.A. and another band at 277 nm with 0.48 U.A., which after the synthesis of the AgNPs move to 218 nm with 2.21 U.A. and the other band at 255.5 nm with 1.33 U.A.
On the other hand, in the visible region, broadband is observed between 323 to 600 nm, centered at 430 nm, corresponding to the surface plasmon generated by the interaction of light and AgNPs (inset of Figure 6). It is observed that the extraction time that allows obtaining the highest amount of AgNPs is 12 minutes, since the highest absorbance is obtained, but then decreases for the 30-minute extract.
Analysis by UHPLC-MS
From the compounds present in the extract, and identified by UHPLC/MS, we can deduce that those with the potential to reduce silver are dihydroxybenzoic acid (4)28 and ellagic acid derivatives (7 and 8).20 Likewise, those compounds that present glycosylated groups in their structure (8, 10 and 11) are possible reducers due to their structural analogy with D-glucose.29 Comparing the UHPLC-MS chromatograms of the aqueous extract of P. racemosa before and after the synthesis of the AgNPs (Figure 4), it is observed that practically all the signals disappear, especially those of tR = 2.28, 2.71, 5.81, 8.75, 23.74 and 25.62 minutes, corresponding to organic acids and some glycosylated triterpenes. As already mentioned, the compounds with the ability to reduce Ag+ ions are compounds 4, 7, 8, 10 and 11. Both the oxidized species of these compounds and the organic acids (including the amino acid) would bind to the nanoparticles to stabilize them, which would explain the disappearance of these signals in the chromatograms.29,30,31,32
Hydrodynamic diameter with DLS
The solutions after the synthesis of the AgNPs were taken to the DLS equipment, where the following results were obtained. Figure 7 shows four volume-weight graphs for the extract readings at different extraction times. It can be seen from the three graphs between 2 and 12 minutes that as the extraction time increases, the relative % of nanoparticles larger than 150 nm decreases. The significant presence of 11.7 nm nanoparticles is also appreciated. In contrast, the 30-minute fraction does not present 11.7 nm nanoparticles, and the relative % of larger nanoparticles increases (22.5 nm and 106.9 nm). Therefore, the extraction time affects the result in the hydrodynamic diameter of the nanoparticles and their percentage distribution in the mixture, the extract being obtained at a time of 12 minutes, with a conductivity of 70 μS/cm and pH 8.2; which allows us to obtain the smallest size of nanoparticles and less polydispersity. Several reports indicate that pH is an important parameter in the stability of AgNPs, indicating that a basic pH allows the formation of small and stable nanoparticles, due to an increase in the reaction rate, with the consequent nucleation of small-sized AgNPs.[33] On the other hand, at pH 8 organic acids are not deprotonated, allowing a greater interaction with AgNPs, preventing their coalescence.
Analysis by scanning electron microscopy
Figure 8 shows a SEM micrograph of the AgNPs obtained with Extract 12. It is observed that the average size of the nanoparticles is around 11.7 nm and they are spherical in shape.
As can be seen from the DLS and SEM results, varying the extraction time of the P. racemosa extract, in addition to modifying the pH and conductivity, also varies the size of the AgNPs. The size of the AgNPs obtained is within the range of values of these nanoparticles obtained by green synthesis. For example, using aqueous extracts of Impatiens balsamina and Lantana camara, AgNPs of 12 nm to 20 nm were obtained for I. balsamina and 3.2 nm to 12 nm for L. camara, depending on the molar concentration of silver nitrate.9 Likewise, when fungi or yeasts are used the size of the obtained AgNPs varies between 5 and 30 nm depending on the species used.11
It is important to mention that the size and shape of AgNPs affect their antibiotic and cytotoxic properties. However, it was observed that there is no linear correlation between size and antibiotic or cytotoxic properties.10 The small size is important for applications and the way they are presented also greatly influences the activity of AgNPs. AgNPs of 89 nm size applied by spray obtained 255 and 4 times more efficacy against S. aureus and C. albicans, respectively. It was also shown that the cytotoxic effect was much lower than the control.12
CONCLUSIONS
The phytochemical study of the aqueous extract of Polylepis racemosa rhytidomes, carried out by UHPLC-MS, has allowed the identification of organic acids, N-acetylglutamic acid, two glycosylated derivatives of ellagic acid (one of them is a new compound, racemosaoic acid) and three glycosylated triterpenes. Despite the relatively few metabolites present in the aqueous extracts, they provided reducing species to obtain Ag(0) and also a capping agent for the stabilization of the synthesized nanoparticles preventing their aggregation.
The extraction time of the aqueous extracts of P. racemosa plays an important role in their physicochemical characteristics and, consequently, in the synthesis of AgNPs. The extract obtained with 12 minutes of extraction allowed us to obtain the smallest AgNPs, with hydrodynamic diameters of 50.8 nm, measured by DLS; while by SEM a diameter of approximately 12 nm was determined. Finally, by comparing the scans by UHPLC-MS before and after the synthesis of the AgNPs, and by the characteristics of the metabolites present, it is deduced that the nanoparticles generated by the reducing groups present were stabilized by the organic acids present in the extract.
The metabolites identified in the aqueous extract of P. racemosa are interesting from the pharmacological point of view, mainly due to the presence of glycosylated derivatives of ellagic acid and rosamultin, which should encourage the phytochemical study of the genus Polylepis, which has not yet received the attention of the scientific community.