INTRODUCTION
Creole cattle from south of Ecuadorian Andean highlands are adapted to harsh environmental conditions (Martínez et al., 2012) including high altitudes, severe air temperature variations and poor-feeding (Aguirre et al., 2011, 2014). Milk production of creole cattle in this region is low and farmers are losing interest in this type of cattle, resulting in the reduction of this population, which may soon can be considered under threat of extinction (Florio & Pineda, 2018). Based on that, the Food and Agriculture Organization of the United Nations - FAO - recommends the preservation of local genetic resources through germplasm banks (HLPE, 2016). Following this recommendation, creole bull semen has been successfully cryopreserved (Argudo et al., 2019). Also, attempts have been made to boost in vitro embryo production (IVP) in creole cows through in vitro maturation (Ayala et al., 2018, 2020; Alvarado et al., 2020), in vitro culture (Méndez et al., 2019) and vitrification of oocytes recovered by transvaginal ultrasound guided follicular aspiration (Méndez et al., 2020). However, the entire IVP process as well as the cryotolerance of creole embryos (e.g., to vitrification/warming) need to be investigated further.
Culture conditions are crucial to assure the quality and quantity of embryos produced in vitro (Rizos et al., 2008). Thus, in vitro bovine embryo production and subsequent cryosurvival after vitrification/warming has been improved by changing the IVC conditions, supplementing the culture medium with additives (e.g., fetal calf serum - FCS) (Sanches et al., 2017), and/or modifying standard cryopreservation procedures (Valente et al., 2022).
Fetal calf serum contains diverse hormones, vitamins, transporting proteins and growth factors that may support early stages of embryo development (Holm et al., 1999; Van Langendonckt et al., 1997). Supplementation of FCS to bovine culture medium enhance number of cells per embryo and blastocysts yielding (Pinyopummintr & Bavister, 1991), and reduce the number of damaged blastocysts cells (Paschoal et al., 2012). FCS exerted a biphasic effect on in vitro embryo development, suppressing very early stages and stimulating subsequent stages of embryo development (Pinyopummintr & Bavister, 1994).
Addition of 0.1, 0.5, 1.0 (Mullaart et al., 2014; Murillo et al., 2017), 2 (Leivas et al., 2011) or 2.5% (Sudano et al., 2011) of FCS to culture medium increased blastocyst production, improving the efficiency of IPV system of bovine embryos. Also, FCS stimulated embryo development from days 4 to 5 of culture (Thompson et al., 1998; Holm et al., 1999) and reduced concentrations (e.g., below 3%, v/v) were useful to sustain embryo quality (Holm et al., 1999). On the contrary, although FCS supplementation to IVC-medium above certain concentrations (>5%, v/v) may improve embryo production, it has been associated with decreased cryotolerance (Rizos et al., 2003; Mucci et al., 2006) and large offspring syndrome in sheep (levels greater than 10%) (Thompson et al., 1995). However, very low concentrations (0.1%) of FCS in culture medium from day 1 to day 5 after IVF resulted in greater early blastocyst rates on day 6 without affecting survival rates after cryopreservation or embryo viability after transference (Murillo et al., 2017). Furthermore, addition of 2.5% FCS in the culture medium did not affect (Paschoal et al., 2012) or slightly reduced (Sudano et al., 2011) the re-expansion rates of the vitrified/ warmed bovine embryos. Recently, it was shown that the addition of 3% FCS on day 5 of culture improved in vitro embryo development but compromised the ability of blastocysts to survive after transfer (Amaral et al., 2022).
It is possible that by reducing the time of embryo exposure to FCS and supplementing 2.5% (v/v) FCS to culture medium on day 5 after IVF, may maintaining the beneficial effect of this protein source on embryo development without affecting subsequent blastocysts cryotolerance. Therefore, the aim of this study was based on two considerations. First, an optimum system for IVP and cryopreservation of embryos from Ecuadorian creole cattle must be established to assure conservation of this genetic resource (Méndez et al., 2020). Second, addition of FCS to culture medium has shown positive effects on blastocyst production (Pinyopummintr & Bavister, 1994; Leivas et al., 2011; Mullaart et al., 2014; Murillo et al., 2017), although its use remains controversial on cryotolerance (Abe et al., 2002; Rizos et al., 2003; Mucci et al., 2006).
Accordingly, this experiment was conducted to assess the effect of supplementing FCS to culture medium on day 5 after IVF on production and subsequent cryotolerance of vitrified/warmed blastocysts derived from Ecuadorian creole heifers managed in a pasture-based system.
MATERIALS AND METHODS
All research procedures were conducted according to the Terrestrial Animal Health Code -2019© OIE (07/08/2019), regarding the use of animals in scientific experiments. Chemicals used in this study were from Sigma Chemical (USA), unless otherwise stated.
Oocytes Collection
Cumulus-oocyte complexes (COCs) were obtained from two oocyte sources in eight collection sessions each: 1) ultrasound guided follicular aspiration from creole heifers and 2) follicular aspiration from abattoirderived ovaries.
The first oocyte source included 10 creole heifers, clinically healthy and aged between 3 to 5 years, which were subjected to ovum pick-up (OPU). Creole heifers grew and reached young adulthood in southern Andean highlands of Ecuador at an altitude above 3500 meters above sea level, and thereafter moved and housed a few months before starting the study at a farm owned by University of Cuenca (Irquis farm) located at an altitude of 2648 m. These animals were managed in a pasture-based system (mix of Lolium perenne, Pemmisetum clandestinun and Trifolium repens), and submitted to periodic deworming, screening diagnostic tests, biosecurity measures and vaccinations to prevent common diseases affecting the herds in that region of Ecuador.
Heifers, which were not hormonally stimulated, were subjected to eight OPU sessions each using an Aloka(R) (Prosound 2, Japan) ultrasound scanner with a 5 MHz sector transducer (UST 9111; Aloka, Japan) attached to the transvaginal guide (WTA, Cravinhos, Brazil). For this purpose, heifers were sedated with 0.05 mg/kg IM of 2% xylazine (Dormi-Xil(R), Agrovet Market, Perú) and applied via epidural 0.3 mg/ml of 0.5% bupivacaine (Bupirop(R), Ropsohn Therapeutics, Colombia). OPU was conducted using an 18 G 1½’’ needle, 75 mm length, connected to the aspiration line and a vacuum pump (WTA BV 003(R), Cravinhos, Brazil) calibrated to 15 mL/min outflow and 70 mmHg. Cumulus-oocyte complexes were recovered into 50 mL conic tubes using VigroTM Complete Flush solution (Vetoquinol, USA) with 10 UI/mL sodic heparin (Sanderson Lab, Chile) pre-warmed at 38.5 ºC as collecting medium. The recovered liquid was washed with phosphate-buffered saline (PBS), filtered, and examined under a stereomicroscope (Nikon, Japan). The OPU were performed at the farm, where the in vitro fertilization (IVF) laboratory is also located.
The second oocyte source included Holstein and Holstein crossbreed ovaries (cows and heifers) collected from the local municipal abattoir. Ovaries were placed in saline solution pre-warmed at 37 ºC and transported within 2 h to the Biotechnology Laboratory of Animal Reproduction in Irquis farm. Cumulus-oocyte complexes (COCs) were recovered by aspiration from 3-8 mm antral follicles using an 18-gauge 1½’’needle attached to a disposable 10-ml syringe. Immediately, COCs were washed in synthetic oviductal fluid buffered with Hepes (hSOF, 107.63 mM NaCl, 7.16 mM KCl, 0.29 mM KH2PO4, 1.7 mM CaCl2 2H2O; 5 mM NaHCO3, 5.35 mM sodium lactate, 4.99 mM Na-pyruvate, 10 mM Hepes acid free, 10 mM Hepes sodium salt, 3 mg mL-1 BSA).
Regardless of the oocyte source, only COCs with three or more compact layers of cumulus cells, smoothly granular oocyte cytoplasm, and no irregularities on the oocyte nucleus were selected for IVM. After collection, both sources of COCs were handled in the same way at all steps of the in vitro embryo production process.
In vitro Maturation
Cumulus-oocyte complexes were cultured (CO2 incubator, Memmert, Germany) in 50 mL droplets (in groups of 25 COCs) covered with mineral oil, in an IVM medium (TCM-199 supplemented with 10% FCS (v/v), 2.2 mg mL-1 sodium bicarbonate, 0.1 mg mL-1 L-glutamine, 0.01 UI mL-1 rh-FSH [Gonal-f, Merck, Italy], 1 µg mL»1 estradiol, 100 µM cysteamine, and 50µg mL»1 gentamicin). COCs were incubated for 24 h at 38.5 ºC under an atmosphere of 5% CO2 in air at maximum humidity. For Creole heifers, COCs from different donors were matured in 50 µL droplets to complete groups of 20-25 structures.
In vitro Fertilization
Matured oocytes were washed twice in IVF-SOF medium (Argudo et al., 2020) (107.63 mM NaCl, 7.16 mM KCl, 0.29 mM KH2PO4, 1.7 mM CaCl22H2O, 25 mM NaHCO3, 5.3 mM sodium lactate, 50 µg mL1 gentamicin, 4.99 mM Na-pyruvat, 0.5 mM fructose, 8 mg mL-1 BSA-FAF, 10 µL mL-1 MEM amino acids, embryo tested ultra-pure water), and transferred to 50 µL droplets (in groups of 25 COCs) of IVF-SOF supplemented with 10 µL mL-1 heparin. Frozen-thawed motile sperm from Bos taurus creole bulls of proven in vitro fertility were centrifugated (10 min at 600 ´ g) on a Percoll discontinuous density gradient (200 µL of 90, 60 and 30% Percoll in a 1.5-mL microtube). Selected sperm were washed in 1 mL of IVFSOF medium by centrifugation (5 min at 200 x g) without heparin and this suspension was added to each fertilization droplet obtaining a final concentration of 1x106 sperm/mL (Day 0). Sperm and oocyte were co-cultures during 18-24 h at 38.5 °C with saturated humidity and 5% CO2.
In vitro Embryo Culture
On day 1 after IVF, cumulus cells were removed from presumptive zygotes by gently pipetting, washed three times in hSOF and transferred to IVC medium (synthetic oviductal ûuid medium modiûed, SOFaaci, as described by Holm et al. [1999]) (107.63 mM NaCl, 7.16 mM KCl, 1.19 mM KH2PO4, 1.51 mM MgSO4 7H2O, 1.78 mM CaCl2 2H2O, 25 mM NaHCO3, 5.3 mM sodium lactate; 50 µg mL-1 gentamicin, 7.27 mM Na-pyruvate, 0.34 mM trisodium citrate, 2.77 mM myoinositol, 10 µL mL-1 MEM amino acids, 30 µL mL-1 BME amino acids, 3 mg mL-1 BSA-FAF, embryo tested ultra-pure water). Putative zygotes were distributed into two experimental groups (with and without supplementation with FCS), and therefore culture was performed in groups no greater than 12 structures in 50-µL droplets covered with mineral oil and incubated (Heracell ™ 150i Tri-gas CO2 incubators, Thermo Fisher, USA) for 6 days at 38.5 °C, in a saturated humidity and atmosphere of 5% CO2, 5% O2, and 90% N2.
FCS Supplementation
After denudation presumptive zygotes were randomly allocated in four groups according to adding or not adding a volume of FCS equivalent to 2.5% of the volume of each droplet to SOFaaci medium on day 5 after IVF: 1) O-FCS+, 2) O-FCS-, 3) AFCS+, and 4) A-FCS-. Control droplets were not manipulated. All embryos were reincubated for 48 h under the same culture conditions described above. On day 7 after IVF, embryos were evaluated morphologically according to Bó and Mapletoft (2013) and categorized classified as morulae (M), early blastocysts (EB), regular blastocysts (RB), expanded blastocysts (ExB) and hatched blastocysts (HB).
Vitrification and Warming
Grade 1 blastocysts from both oocytes source (OPU and abattoir) were subjected to vitrification and warming in eight replicates. Briefly, blastocysts were washed in holding solution (HS = hSOF + 20% FCS) and transferred to 500 mL vitrification solution 1 (VS1; HS + 7.5% ethylene glycol + 7.5% dimethyl sulfoxide [DMSO]) for 3 min. Then, embryos were placed into 50 mL droplets of vitrification solution 2 (VS2; 0.5M sucrose + 16.5% ethylene glycol + 16.5 DMSO) for <30 s (VS1 and VS2 were elaborated with hSOF). Thereafter, embryos were loaded into open pulled straws (OPS) in a volume of 1 mL of VS2 and submerged into liquid nitrogen for 90-120 days.
Warming was performed by placing the narrow end of the OPS into four-well dish with 800 mL of 0.5 M sucrose in 20% FBS solution (WS1) for 5 min. Next, embryos were transferred into a 400 mL droplet of 0.25 M sucrose solution (WS2) for 5 min (WS1 and WS2 elaborated with hSOF), washed twice in hSOF, and then incubated in SOFaaci supplemented with 20% of FBS for 48 h at 38.5 °C in a humidiûed atmosphere (5% CO2, 5% O2 and 90% N2). Cryotolerance of vitrified/warmed embryos was evaluated according to blastocele re-expansion and subsequent hatching at 2, 24 and 48 h postwarming.
Statistical Analysis
To compare proportions of cleavage, embryo (morula and blastocyst) and blastocyst (early blastocysts, regular blastocysts, expanded blastocysts and hatched blastocysts) on day 7, and blastocysts re-expansion and hatching between experimental groups, data were analyzed by binary logistic regression of SAS v 9.3 (SAS Institute, USA). Effects of oocytes source, FCS supplementation, replicates (n=8), and oocytes source ´ FCS supplementation interaction were considered as independent factors in the statistical model. Contrast test was used to determine differences between oocytes source and FCS supplementation. P values between 0.051 and 0.1 were interpreted as a tendency.
RESULTS
According to oocytes source, rate of cleavage, morula/blastocyst yield and developmental stages of embryos on day 7 was similar between OPU and abattoirderived ovaries (Table 1). There were no statistical differences in rates of re-expansion (2, 24 and 48 h) and hatching (24 and 48 h) between oocytes source (data not shown).
Table 1. Rate of cleavage, embryo production and developmental stage of embryos on day 7 after IVF according to oocytes source

OPU: oocytes recovered by ovum pick-up; Abattoir: oocytes recovered from abattoir-derived ovaries
M: morulae; EB: early blastocysts; RB: regular blastocysts; ExB: expanded blastocysts; HB: hatched blastocysts
Regardless of oocyte source, greater proportion of embryos from FCS+ developed on day 7 than FCS-(P = 0.0871), although rate of blastocysts did not differ between groups (Table 2). Following vitrification/ warming, a greater proportion of blastocysts from FCS-re-expanded at 2 h of culture than FCS+ (p<0.01). Rate of re-expansion at 24 and 48 h and hatching at 24 h did no differ between groups; however, blastocysts from FCS-had greater hatching rate at 48 h than those from FCS+ (p<0.01) (Table 2).
Table 2. Total embryos and blastocysts on day 7, and blastocysts cryotolerance according to addition of fetal calf serum (FCS) to culture medium on day 5 after in vitro fertilization (IVF)
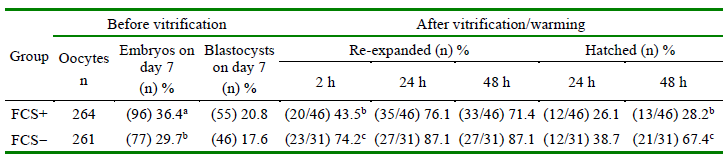
Group supplemented with 2.5% (FCS+) or 0% (FCS-) of fetal calf serum in culture medium on day 5 post-IVF. Q1= Blastocyst of quality 1 subjected to vitrification. Values with different letters in the same column differ: a-b P = 0.0871; b-c P < 0.01
Data of in vitro embryo production and cryotolerance according to whether 2.5% of FCS was added to culture medium on day 5 after-IVF, for each oocyte sources are shown in Table 3. For both oocyte source, total embryo and blastocysts rates on day 7 did not differ between FCS groups (p>0.05). After vitrification-warming, however, addition of FCS to culture medium at day 5 after IVF affected blastocyst re-expansion only at 2 h of incubation, irrespectively of oocyte source, with lower percentages in O-FCS+ and AFCS+ than in O-FCS-and A-FCS-groups (p<0.05). Although differences were not significant, re-expansion rate of blastocysts at 24 and 48 h was over 20 percentage points in O-FCS-vs O-FCS+ group. Likewise, hatchingrate of blastocyst at 48 h of incubation was drastically affected by vitrification only in oocytes from OPU-derived ovaries with a 59.6 percentage points greater in O-FCS-than in O-FCS+ (p<0.01) (Table 3). In addition, rate of hatching was 22.6 percentage points greater in A-FCS-than in A-FCS+ ovaries.
Table 3. Embryo in vitro production and cryotolerance of creole cattle blastocysts according to oocytes sources and addition of fetal calf serum (FCS) to culture medium on day 5 after in vitro fertilization (IVF) (n = 8 replicates)
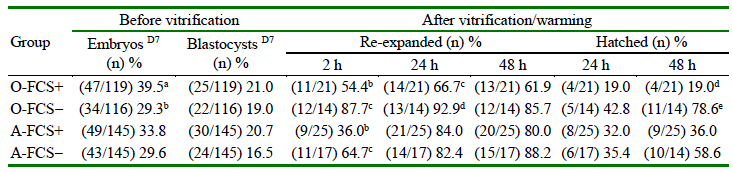
Group supplemented with 2.5% (FCS+) or 0% (FCS-) of fetal calf serum in culture medium on day 5 after IVF
O: OPU; A: Abattoir. D7= Day 7
Values with different letters in the same column for each oocyte source differ: a-b p = 0.07; b-c p<0.05; c-d p = 0.067; d-e p< 0.01
DISCUSSION
According to findings is feasible to produce genetic material from Ecuadorian Creole heifers for transference of fresh in vitro derived embryos. Nevertheless, FCS added to IVC-medium on day 5 after IVF reduced blastocyst cryotolerance compared with embryos no exposed to FCS. Whereas FCS improved blastocysts production, these embryos were more sensitive to vitrification and fewer of them achieved hatching rates comparable to those developing without FCS in the culture medium (Abe et al., 2002; Mucci et al., 2006; Rizos et al., 2003). In these studies, the culture medium was supplemented with 5% or more FCS 24-48 hours after IVF; so that embryos were exposed to FCS for at least 120 h. Adding 2.5% FCS on day 2.5 of culture increased blastocyst production and reduced rate of reexpansion of vitrified blastocysts compared to control, without affecting embryo cell number neither rate of apoptosis (Sudano et al., 2011).
Based on this evidence it is assumed that reducing the period of embryo exposure to FCS to 48 h and decreasing the percentage of proteins to 2.5% might improve blastocyst production without affecting tolerance to vitrification, ensuring an efficient in vitro system for preservation of the genetic material. However, addition of 2.5% FCS to culture medium at day 5 after IVF did not improve embryo production and negatively affected subsequent blastocyst cryotolerance, particularly in those from heifers.
In this study there was no effect of the oocyte source on the proportions of different developmental stages of embryos evaluated on day 7 after IVF. Several studies compared the development capacity of oocytes collected by OPU or from abattoir-derived ovaries and the results were contrasting. For instance, a greater proportion of OPU-derived oocytes reached the morula/blastocyst stage at day 7 in cows (Karadjole et al., 2010) and buffalos (Neglia et al., 2003) compared with abattoirderived oocytes. In other studies, no differences between both oocyte sources were found in terms of embryo production (Galli et al., 2001), or proportionally more blastocysts were yielded from abattoirderived oocytes than from OPU (Quispe et al., 2018). Previously, this group reported that oocytes obtained by OPU from Creole heifers were 3.3 mm smaller, a slighter proportion had the plasma membrane intact and a lesser number of them were classified as class 1 and 2 oocytes compared to those obtained from abattoir ovaries (Alvarado et al., 2020). Hence, oocyte competence to reach blastocyst stage after IVF might be affected in OPU-derived oocytes from Creole heifers reared under the environmental and altitude conditions of this study.
Regardless of the source of oocytes, addition of 2.5% FCS to the SOFaaci medium on day 5 after IVF increased ~7% extra to morula/blastocyst production without affecting blastocyst rates. Moreover, in OPU-derived oocytes rate of morula/blastocysts were around 10% greater in O-FCS+ than in O-FCS-. These findings are comparable with others. Thus, percentages of FCS as low as 0.1% were effective to improve blastocyst yield and increased the efficiency of the in vitro bovine embryo systems (Mullaart et al., 2014). Abdel-Wahab et al. (2018) showed that both newborn calf serum and FCS stimulated morula/blastocyst yield when added on day 1 of culture. Supplementation of FCS on day 4 after IVF decreases the period of exposure to serum and had a favorable effect on quality 1 blastocyst production (Leivas et al., 2011).
Nevertheless, after vitrification/warming of day-7 blastocysts, FCS supplementation decreased rates of blastocyst re-expansion at 2 h of incubation in both oocyte sources. Interestingly, at 48 h of re-incubation FCS negatively affected rate of hatching of both oocytes source, with a significant greater magnitude in embryos from OPU-derived oocytes. The ability of in vitro produced embryos to survive cryopreservation, an indicator of embryo quality, is affected by culture conditions (Costa et al., 2005; Rizos et al., 2003). Cryotolerance of bovine embryos is affected by lipid accumulation (de Andrade Melo-Sterza et al., 2021), which apparently occurs due to adding FCS in the culture media (Sudano et al., 2011). Bovine embryos cultured in FCS-containing medium abnormally accumulated cytoplasmic lipids and this excessive amount of lipids affected embryo cryotolerance (Abe et al., 2002). Embryos cultured with 2.5% FCS plus PES (phenazine ethosulfate, inhibitors of fatty acid synthesis) slightly increased the lipid content compared to those cultivated in FCS-free medium.
The current study reveal that 2.5% FCS added to the culture medium on day 5 after IVF affected drastically the cryotolerance of blastocysts derived from creole heifers. It is plausible that genetic characteristics of Creole bovines may have influenced embryo cryotolerance. Sudano et al. (2014) indicated that the breed may change the cryotolerance response of bovine embryos, as differences have been reported in the content of intracytoplasmic structures (e.g., mitochon-dria, lipid droplets, endosomic cistern vesicles) between Bos taurus and Bos indicus breeds (Visintin et al., 2002). Significant differences in lipid content were demonstrated between embryos from Nellore and Simental breeds, with less abundance of cytoplasmic lipids in embryos from the former than the latter breed (Sudano et al., 2012). Interestingly, although the blastocyst rate was significantly greater in Nellore than in Simental cows, a greater proportion of blastocysts from the latter reexpanded and hatched more than the former (Sudano et al., 2014), demonstrating a greater cryotolerance of Simental embryos, which according to evidence had greater content of intracytoplasmic lipids.
On the other hand, another aspect to be considered to assess embryo cryotolerance of Creole heifers is the quality of oocytes of this genetic group. As mentioned above, in a previous study (Alvarado et al., 2020) morphological and functional differences were demonstrate in oocytes from Creole heifers that may have implications in subsequent embryonic development and cryotolerance, and therefore, in the success of preserving this valued genetic material. For instance, plasma membrane integrity of oocytes from Ecuadorian creole heifers recovered by OPU was significantly lower than those obtained from abattoir-derived ovaries (Alvarado et al., 2020). Accordingly, embryos originated from creole heifers might be more sensitive to cryopreservation, likely because of the lower integrity of the oocyte plasma membrane.
An additional important factor to consider is the high altitude at which creole heifers were raised. Life at high altitude has the potential to adversely affect some bodily functions according to whether individuals are adapted or not. Low atmospheric oxygen pressure and temperatures, increased exposure to solar radiation, and greater subsistence energy consumption may potentially affect diverse physiology functions (Little & Hanna, 1978; West, 1999; Beall, 2006). Is known that hypobaric hypoxia originated by high altitude acts as a stressor that reduces fertility (Vargas et al., 2011). Living at high altitudes disrupts several aspects of the reproductive function (Parraguez & Gonzalez Bulnes, 2020) and decreases fertility in humans (Verratti & Di Giulio, 2012) and in sheep (Parraguez et al., 2013) compared to their equals living near sea level. Thus, it is plausible that the altitude at which this experiment was conducted and at which these criollo females were reared, coupled with the effect of FCS, may have influenced blastocyst production and the tolerance of creole embryos to vitrification.