Introduction
Biological invasions are one of the main concerns for the conservation of ecosystems since they can lead to the replacement of native by non-native species (Levine 2008, Simberloff et al. 2013) and constitute one of the main causes of biodiversity loss (Drake & Lodge 2004, Shuai et al. 2018). The invasive exotic species can radically change both the species composition and functioning of native ecosystems, modifying the interactions of the native biota, directly as well as indirectly. And may even threaten human health (e.g. parasites co-introduced with the exotic species) (Levine 2008, Makowski & Finkl 2019, Šimková et al. 2019). They are the cause of dramatic changes related to the abiotic characteristics, biological and ecological aspects, and vulnerability of the colonized community (Pérez et al. 2006, Catford et al. 2009, Shuai et al. 2018). These impacts usually occur with high rates of reproduction and spread of the exotic species, combined with the absence of predators and considerable plasticity to the new environment (Katsanevakis et al. 2014). However, factors such as climate change and impacts related to human population explosion and its activities, including aquaculture and maritime transport, are considered responsible forces for the intensification of biological invasions worldwide (Pierucci et al. 2019).Generally, the early stages of these biological invasions (associated with introduction, establishment, and initial dissemination) are not fully documented (Puth & Post 2005) when compared to the last stage of invasive dissemination where impacts begin to appear (Blackburn et al. 2011).
The genus Mulinia currently comprises nine species widely distributed in the Pacific and Atlantic oceans along the coast of North, Central, and South America. Several species occur between the two oceans, in the Strait of Magellan, a passage located between Chilean Patagonia, Tierra del Fuego Island, and other islands in this region (Signorelli 2019). In the Pacific coast of south America, the genus Mulinia are represented by five species; M. edulis (King, 1832) distributed from Guayaquil in Ecuador to the Strait of Magellan and Tierra del Fuego in Argentina; M. pallida (Broderip & Sowerby, 1829) distributed from the Gulf of California in Mexico to Peru; M. byronensis Gray, 1837 distributed from Peru to Argentina (Ushuaia); M. exalbida Gray, 1837 distributed from Chile (Concepción) to Magellan Strait; M. bicolor Gray, 1837 from Chile (Talcahuano); and M. levicardo (Smith, 1881) from Magellan Strait (Chile) (Signorelli 2019).
In 2018, during a visit to a shrimp farm of whiteleg, Penaeus vannamei (a decapod crustacean native to the eastern Pacific coast from northern Mexico to Tumbes in Peru, and of great economic importance to Ecuador), we could confirm the abundant presence of a species of bivalve covering the bottom of the pools (Fig. 2). The farm worker in charge of handling these shrimp pools informed us that since a couple of years ago, they have been observing this situation that has become a problem because the filtration of these molluscs is so aggressive that they consume almost all the phytoplankton of the ponds, which also brings problems with dissolved oxygen due to the lack of photosynthesis because of the absence of microalgae, and the consumption of this gas for the breathing of bivalves. The clearing of the water column in the ponds also increases the exposure of shrimps to predatory birds.
This study reports for the first time, the presence of a population of Mulinia lateralis (Say 1822) on the coast of southern Ecuador, a species that occurs naturally in the Atlantic Ocean and it has been suggested as possible successful invader in Europe (Craeymeersch et al 2019). Morphological characteristics of the species and the confirmation of its identity by DNA barcode technique are provided.
Material and methods
Taxon sampling and morphological analysis. The bivalves were sampled from a shrimp farm located in Estero Quiriquinchal, Jambelí Archipelago, Santa Rosa City, El Oro province, Ecuador (3°15'39"S, 80°01'41"W), between the years 2018 and 2019. The species was identified based on morphology according to Say (1822); Signorelli (2019); Signorelli and Pastorino (2011, 2012); Craeymeersch et al. (2019). The samples were preserved in absolute ethanol at -20 °C until molecular analysis. Voucher specimens collected at 15 july 2019 are deposited in the collecction of the Universidad Técnica de Machala at the numbers UTMACH0 454, UTMACH0 455, UTMACH 0456.
DNA extraction, PCR, and sequencing. Total DNA was extracted from the ethanol-preserved adductor muscles using the Wizard Genomic DNA Purification Kit (Promega, Madison, Wisconsin, U.S.A.) following the manufacturer’s recommendations. A partial sequence of mitochondrial genes COI was amplified using polymerase chain reaction (PCR) with the following primers: ZplankF1 and ZplankR1 (Prosser et al. 2013). The PCR product was loaded into a reaction of 12.5 μl, containing 1.25μl of 10 X PCR buffer, 0.25 μl of MgCl2 (50 Mm), 0.2 μl dNTPs (2 mM), 0.5 μl of each primer (5 mM), 0.1 μl de 1.25 U of pht DNA Taq polymerase, and 1 μl of genomic DNA (200 ng). The PCR cycles were done under the following specifications: 94 °C x 5 min, 30 cycles of 94 °C for 40 s, 50 -52 °C for 30s, 68 °C for 1 min, and 72 °C for 8 min. The PCR products were checked on an agarose gel at 1%. All PCR products were purified and sequenced in both directions by Macrogen Inc. (Seoul, South Korea).
Sequence alignment and phylogenetic analyses. To assemble and edit the contigs, the software in Geneious v5.5.6 (Drummond et al. 2011) was used. Alignment was performed using the Muscle algorithm under predetermined parameters (Edgar 2004). To calculate the genetic distance, the Kimura-2-parameter model was used, including the bootstrap analysis (Felsenstein 1985) with 1000 nonparametric bootstrap replicates, using the MEGA X software (Kumar et al. 2018). Maximum likelihood (ML) analysis was performed using RaxML Web-Servers Black-Box (Stamatakis et al. 2008) with GTR + G + I as a model. Random starting trees were used for each independent ML tree search, and all other parameters were set to default values on the server. Topological robustness was investigated using 1000 nonparametric bootstrap replicates (Felsenstein 1985). The COI gene sequence of Mulinia lateralis from Ecuador was compared with sequences of M. lateralis from the Atlantic Ocean, M. edulis, M. pallida, and Potamocorbula amurensis (Schrenck 1861) as an outgroup. All sequences were retrieved from Genbank and Bold system (Table 1).
Results
Morphologic characteristics. The specimens herein studied were identified as Mulinia lateralis (Fig. 1). They were all small to medium size (~15 mm mean ± standard deviation: 14.94±0.53; n=22); the shells were equilateral (Fig. 1) and exhibited a rounded and trigonal form, with prominent umbos in subcentral position, from where radial ridges appeared in the posterior side of the valve; external shell with periostracum colour cream to white-yellowish and a surface smooth with very fine concentric growth lines. Ligament exclusively internal; lateral anterior teeth in the right valve of different sizes, the ventral one longer and two lateral posterior teeth similar in size, with the chondrophore triangular and slightly ventrally projected.
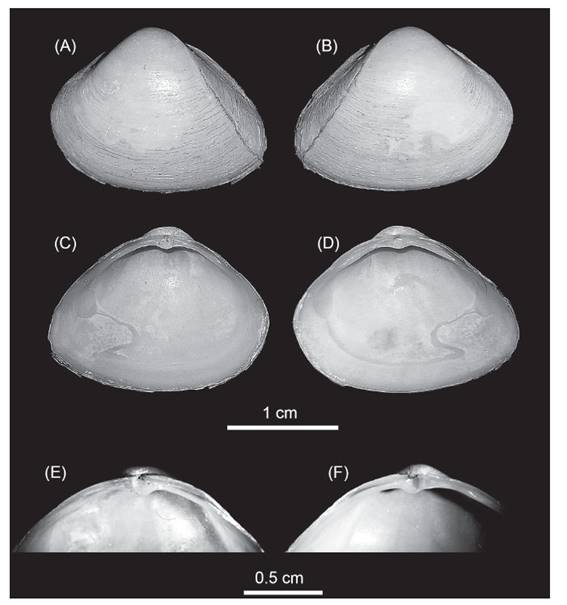
Figure 1 Mulinia lateralis, Ecuador, El Oro province, Santa Rosa City, Jambeli Archipelago, Estero Quiriquinchal. A, C, E: left valves; B, D, F: right valves; E, F: hinge teeth detail.
These characteristics are concomitant with Mulinia lateralis (Say, 1822), a species with an Atlantic Ocean distribution from Canada to Central America (Signorelli & Pastorino 2011, 2012, Craeymeersch et al. 2019, Signorelli 2019). Others species of the genus Mulinia that have been reported from the Ecuadorian coast, are Mulinia edulis and Mulinia pallida. Both species are very different from M. lateralis; Mulinia edulis is subcircular in shape with more inflated and prosogyrous umbos, its periostracum is reddish brown, and Mulinia pallida has a subequilateral shell with a sub-trigonal form and an elongation towards the posterior area; the periostracum is yellowish (Signorelli 2019).
Genetic data. Sequences obtained in this study were deposited in GenBank and Bold system under accession numbers provided in Table 1. The sequence data set resulted in a matrix with 647 aligned base pairs (bp), of which 242 characters were variable and 228 were parsimony informative. The estimated index of substitution saturation (Iss) performed in DAMBE 5.2.31 (Xia & Xie 2001) showed that the data were not saturated (i.e. Iss.c value greater than Iss). Genetic interspecific distances (Kimura 1980) of the COI gene between Mulinia lateralis from Pacific Ocean (Ecuador) and M. lateralis from Atlantic Ocean (FL-MA, USA) was 0.008 ± 0.004; between M. lateralis from Pacific Ocean (Ecuador) and M. lateralis from Wadden Sea (Netherland) was 0.004 to 0.008 ± 0.004 to 0.006; between M. lateralis from Pacific Ocean (Ecuador) and M. edulis from Pacific Ocean (Chile) was 0.150 ± 0.018; between M. lateralis from Pacific Ocean (Ecuador) and M. pallida from Pacific Ocean (Peru) was 0.139 ± 0.017, and finally between M. lateralis from Pacific Ocean (Ecuador) and Potamocorbula amurensis from Pacific Ocean (China) was 0.385 ± 0.032 (Table 2). The cladogram (Fig. 3) indicated three clades strongly supported by a high bootstrap index (52 - 100%) of the genus Mulinia, the first clade grouped the individuals of M. lateralis from Ecuador, the USA, and The Netherlands, differentiating them from the other species analysed.
Discussion
Only two species of the genus Mulinia have been reported from the Ecuadorian coast, Mulinia edulis from Guayaquil in Ecuador to Ushuaia in Argentina, and Mulinia pallida from the Gulf of California in Mexico to Peru, both are larger organisms with a strong shell and different morphological characteristics.
The morphological characteristics of the analysed individuals from Ecuador clearly coincide with the characteristics of the species originally reported as M. lateralis sensu Signorelli (2019) and differ from those of M. edulis and M. pallida. Likewise, there are genetic differences between M. edulis and M. pallida as mentioned in genetic data section. In addition, the genetic sequences of M. lateralis collected in Ecuador present a high genetic similarity of 99.83% and 99.35% (BOLD and BLAST, respectively) with those sequenced from the USA and The Netherlands, confirming the species, and differentiating it from the other Mulinia that inhabit the Pacific Ecuadorian coast.
Mulinia lateralis is a benthic bivalve found on soft substrates, where it normally remains buried (Levinton 2001). Adults measure 15 to 20 mm in length, while shells of newly metamorphosed juveniles measure 200 to 700 µm (Wang & Guo 2008). They have separate sexes and reach sexual maturity in just 60 days, with an average life span of up to two years (Guo & Allen 1994, Lu et al. 1996).
In the shrimp farm, all bivalves were observed in the estuary and the pools. In the study area, M. lateralis is quite common, especially in the winter months (May to September), while in the summer months (November to March), it disappears, leaving only its shells at the bottom of the ponds. From 10 samples randomly obtained at different points in the pool, using a 0.25 m2 quadrant, an average density of 1977 ± 487 ind./m2 was estimated (Fig. 2), although it has been reported that it can reach a density of more than 21,000 ind./m2 after catastrophic events (Santos & Simon 1980), which is an indicator of the reproductive capacity of this invasive bivalve.
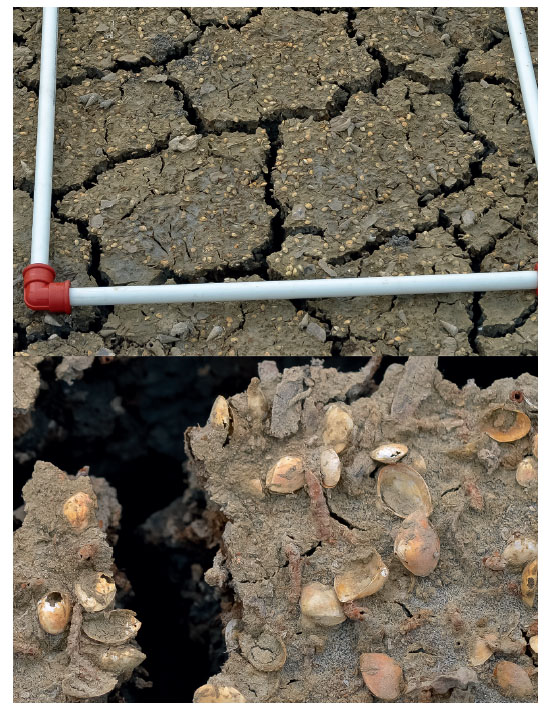
Figure 2 Abundant presence of Mulinia lateralis covering the bottom of the pools in the shrimp farm from Estero Quiriquinchal, Jambelí Archipelago, Santa Rosa City, El Oro province, Ecuador, February 28, 2018.
Mulinia lateralis is considered by many studies as an opportunistic species, with a very short generation time (six weeks at 25 °C), where its occurrence is characterized by the rapid appearance of newly installed juveniles on the bottom, and later large sudden mortalities, leaving large deposits of dead shells (Calabrese 1970, Grassle et al. 1992).
It is also important to point out that, based on the information provided by the staff of the shrimp company where the M. lateralis specimens were collected, the presence of the bivalves in the culture ponds altered the behaviour of the shrimp. Due to the clearing of the water in the ponds through the active filtration by the bivalves, the water with little turbidity favoured the attack of the birds during the day, forcing the shrimp to remain on the bottom. Records of ponds’ water conditions indicated a decrease in total alkalinity, which could be attributed to the explosive presence of bivalves that consumed much of the CaCO3 to build up their shells, limiting the availability for the shrimp. The staff that managed the culture ponds also informed us of the presence of diseased shrimp more frequently than usual. Since the low alkalinity caused by the reduction of CaCO3 also affects the pH, it is possible that this parameter has been a stress factor in the shrimp, promoting the appearance of diseases.
An important aspect of the invasion process is the ability of invader species to overcome natural biogeographic barriers, as a result of intentional human transport or colonization routes created by anthropic activity (Rahel 2002; Trakhtenbrot et al. 2005; Mohanty & Measey 2019). Indeed, the main source of marine introductions globally is ballast water discharge from international shipping and fouling of commercial ship hulls which constitutes a major threat to the oceans, generating international concern for environmental, health, and economic reasons. The problem worsens in places where the ballast water discharge is not regulated since it is estimated that around 7000 species (larvae and small fish, crustaceans, algae, invertebrates, bacteria and viruses) are transported around the world via ballast water every day.
Several studies have shown that exotic macroinvertebrates, particularly bivalve, and decapod larvae, are the most commonly transported organisms in ballast tanks or connected to ship surfaces (Karatayev et al. 2009, Briski et al. 2010, 2012, Pejovic et al. 2016). Introduced bivalve molluscs may change the benthic community and be direct competitors of native species (Ward & Ricciardi 2007), while crustaceans affect bivalve molluscs and other crustaceans through mainly predation and competition, causing great economic losses (Briski et al. 2012).
In Ecuador, ballast water discharge at its main harbours was not regulated until 2017 (Grau Ávila 2018). Several small bivalve molluscs (clams, mussels, snails) were introduced mainly in the Gulf of Guayaquil, and currently have become pests in the soils of the shrimp farms, because they have a great filtration capacity, reduce the concentration of plankton, organic matter, oxygen, and calcium carbonate, and also cause water clearance in ponds of shrimp farms, which can lead to the production collapse (Torres Chuquimarca 2012). One such example of other species introduced in Ecuador is Potamocorbula amurensis (Schrenck 1861). It is a bivalve mollusc native to Siberia (Russia), China, Korea, and Japan; which has also been reported in the Bay of San Francisco (USA) since 1986 (Carlton et al. 1990) and was also reported in the Gulf of Guayaquil, Ecuador, in 2012, by Torres Chuquimarca (2012). This author attributed its presence to the ballast water discharge from commercial vessels in the harbours of the Gulf of Guayaquil.
Regarding M. lateralis, the molecular data and intraspecific genetic distance (Fig. 3 and Table 2) show the higher genetic affinity between the samples from Florida with the sample from Ecuador and the Netherlands, compared with samples from Maryland (USA), which leads us to suppose that M. lateralis could have reached the region of Puerto Bolívar (Ecuador) from the port of Tampa Bay, Florida (USA). Considering that this species has established populations in estuarine areas of Puerto Bolívar (Ecuador) and has the potential to become a successful bioinvasive organism, it is likely that it will spread to other areas of Ecuador. This study, in addition to constituting the first report on the presence of M. lateralis in Ecuador, is an alert so that the institutions responsible for ensuring the administration of biodiversity adopt precautionary measures to control and/or eradicate this invasive species so as not only avoid affecting aquaculture farms in Ecuador but also protect the aquatic biodiversity of the region.