1. Introduction
Agricultural crops require chemical fertilizers to supply the necessary nutrients for their development (Rouphael & Colla, 2020); however, the apparent recovery efficiency under favorable conditions for nitrogen (N) from fertilizer is 50%-70%, for phosphorus (P) 10%-25% and for potassium (K) 50%-60% (Ahmed et al., 2017; González et al., 2016). Urea is the main source of applied nitrogen, being converted to ammonia in the soil that can be volatilized or oxidized to nitrate. This compound can be lost by processes such as leaching, denitrification and runoff (Timsina, 2018). Phosphorus is one of the essential macronutrients for plant growth; however, the concentration of this soluble element is very low in the soil (400-1260 mg/kg), and the application of chemical fertilizers such as triple superphosphate, diammonium phosphate and ammonium polyphosphate is usually required (Joe et al., 2018); however, more than 85% of the applied phosphorus comes from the non-renewable resource phosphate rock (Ahuja et al., 2020).
Liquid organic fertilizers such as biofertilizer from the anaerobic fermentation of residues such as fish are low cost and easily available. This organic fertilizer contains microorganisms (Hernández-Álvarez et al., 2023) and necessary nutrients for agricultural crops (Espinales et al., 2023; Maroulis et al., 2023), which has been tested with positive results related to the increase in height, flowering days, number of branches, fruit weight and yield of Capsicum annuum “pepper” (Salavarría, 2023), height, root length, biomass and yield of Oryza sativa L. "rice" (Saputra et al., 2022; Kusuma et al., 2021), fruit yield of Lycopersicon esculentum var. Cerasiform "cherry tomato" (Choi, 2020), germination percentage of Lactucca sativa "lettuce" seeds (Florez et al., 2020) and height, number of leaves and root weight of Raphanus sativus "radish" (Acosta, 2019); however, those biofertilizers whose characteristics have not been correctly evaluated could contaminate the environment and be a risk to human health due to the presence of pathogens or heavy metals (Chuan et al., 2017). Moreover, it is necessary to investigate its properties at the field level prior to its application in crops.
For all these reasons, the aim of this study was to evaluate the impact of a biofertilizer from fish wastes on the yield of important crops such as Vigna unguicuata L. Walp "cowpea" and Zea mays L. "corn", as well as its effect on rhizospheric microorganisms associated with these crops.
2. Methodology
2.1. Population and sampling
The biofertilizer from fish wastes was obtained from the Association of Artisanal Processors of Hydrobiological Products of the Santa Rosa Fishing Center "APAPHCEPES", in Lambayeque, Peru. In the preparation of the organic fertilizer, derivatives from the gutting of cured fish (stomach, intestine, liver, heart, blood, gonads and fish derivatives) of the species Scomber japonicus peruanus “mackerel”, Sarda chiliensis “bonito” and Mugil cephalus “flathead grey mullet” were used. The proportions of the materials used were: 10% molasses, 10% Gar-Lac microbial consortium, 40% eviscerated derivatives and 5% hydrobiological waste (Suárez et al., 2022).
Two independent trials were carried out on the crops of Vigna unguicuata L. Walp "cowpea" and Zea mays L. "corn", under a completely randomized blocks design (RCBD), with six treatments, three blocks and 18 experimental units per crop. In each trial, the treatments were: absolute control (T1), chemical control (T2), biofertilizer 1% (T3), biofertilizer 1.25% (T4), biofertilizer 1.5% (T5) and biofertilizer 1.25% plus chemical fertilizer (T6).
2.2. Physicochemical and microbiological characteristics of the biofertilizer from fish wastes
The physicochemical characterization of the biofertilizer was carried out in the Soil and Water Laboratory (LABSAF) of the National Institute of Agrarian Innovation (INIA), while the microbiological analysis was carried out according to the Peruvian Technical Standard NTP 360.506.2020 (INACAL, 2020).
On the other hand, phytotoxicity tests were carried out using Raphanus sativus L var. Crimson Giant “radish” seeds and Allium cepa “red onion” seed-bulbs. For this, seed germination was carried out in Petri dishes conditioned with absorbent paper moistened with 10 mL of three dilutions of biofertilizer (10-1, 10-2, 10-3), using saline solution (NaCl 0.85% w/v, 75% humidity) as a control, corresponding to four treatments, three repetitions per treatment, and 20 seeds per repetition. After 120 hours, the germinated seed count and germination index (GI) were performed (Lakhal et al., 2017).
The phytotoxicity level was established considering the relationship between the GI and the number of phytotoxic substances: GI ≥ 80% (absence or very low number of phytotoxic substances - low level), 80% > GI > 50% (moderate presence of phytotoxic substances - moderate level), GI ≤ 50% (strong presence of phytotoxic substances - severe level).
The phytotoxic effect of biofertilizer on the growth of Allium cepa seed-bulbs roots was determined in beakers with 70 mL of three decimal dilutions of biofertilizer (10-1, 10-2, 10-3) in dechlorinated drinking water for 24 hours, 70 mL of dechlorinated drinking water as the first control and 70 mL of a copper sulfate solution (CuSO4, 0.25 g/L) as the second control, corresponding to five treatments, twelve repetitions per treatment, and one seed-bulb per replicate. After 120 hours, the minimum and maximum values in the length of the roots were determined, and the mean value observed in most of the roots was recorded. In each treatment, twelve values of root length were recorded, the highest and lowest values were eliminated, and the remaining ten values were averaged to calculate the percentage of inhibition with respect to the first and second control (Benavides & Plasencia, 2012).
2.3. Effect of biofertilizer on the growth and yield of Vigna unguiculata L. Walp and Zea mays L.
The effect of three doses of the biofertilizer from fish wastes on the growth and yield of Vigna unguiculata L. Walp "cowpea" and Zea mays L. "corn" was determined in two independent trials for each crop, with an experimental area of 317.63 m2 per crop in Lambayeque, Perú (Latitude: -6.63, Longitude: -79.80), from February to May 2022, with an average temperature between 22-26 °C, relative humidity of 71% and rainfall between 0-2 mm (SENAMHI, 2022).
2.3.1. Physicochemical and microbiological analysis of the experimental soil
Using the quartering and piling method (Contreras & Carreño, 2018), a representative sample of 0.1 kg of soil was collected for microbiological analysis and 1 kg for physicochemical analysis. According to the physicochemical analysis, the soil of the cowpea crop was potentially sodium saline, with an electrical conductivity of 10.08 dS/m, moderately basic (pH 7.85), low content of organic matter (1.2%) and phosphorus (6.7 ppm), and average potassium content (120 ppm). The soil of the corn crop was potentially sodium saline, with an electrical conduc tivity of 11.32 dS/m, moderately basic (pH 7.65), low organic matter content (0.7%), and medium content of phosphorus (25 ppm) and potassium (117ppm).
The microbiological analysis consisted of counting nitrogen-fixing and phosphate-solubilizing bacte ria. In each rhizospheric soil sample, six consecutive decimal dilutions were made starting from 10 g of soil, the 10-4, 10-5 y 10-6 dilutions were selected, and 0.1 mL of each of these dilutions was deposited in the center of Petri dishes with nitrogen-free (NF) agar for the count of nitrogen-fixing bacteria, and with National Botanical Research Institute Phosphate (NBRIP) agar for the count of phosphate solubilizers, incubating them at 30 °C for 7 days. In the NF agar, the Colony Forming Units (CFU) present in two consecutive dilution plates with 30-300 CFU were counted, while in the NBRIP agar, the CFUs were counted in the highest dilution plate with solubilization halos (Córdova et al., 2022; Altamirano et al., 2021).
2.3.2. Cultivated plant species
Seeds of Zea mays L. "corn" hybrid DeKalb - 7508 and Vigna unguicuata L. Walp "cowpea", variety INIA 432 - Green Pod, which are marketed previously treated with the fungicide Captan 200 g/L plus Carboxin 200 g/L (Vitavax), were used in this study.
2.3.3. Establishment and management of cowpea crop
Three cowpea seeds were sown by blow to a depth of 5 cm. A 9-hour irrigation (divided into 3 consecutive days) was applied immediately after sowing (germination irrigation), five irrigations (4 hours each) before flowering, and two irrigations (4 hours each) during fruiting. To control weeds, two hand controls were carried out at 17 and 40 days after sowing.
During the development of the crop, the control of Empoasca kraemeri "green parrot", Alpis fabae "black aphid" and Fusarium sp. was made applying Imidacloprid (INTOCABLE 70WP, Yixing xingnong chemical products, China) at a dose of 700 g/ha plus Tebuconazole and carbendazim (ESPOLON, Anasac crop science, China). In addition, yellow flags with TEMO-O-CID (Kollant S.R.L, Italia), an adhesive for the ethological control of flying insects, were placed.
2.3.4. Establishment and management of corn crop
Corn was cultivated by dry sowing, using the sowing system by blow depositing three seeds at a depth of 8 cm. A 9-hour irrigation (divided into 3 consec utive days) was applied immediately after sowing (germination irrigation), and then the irrigations were twice a week for 4 hours each irrigation. For weed control, two hand controls were carried out at 16 and 40 days after sowing.
During cultivation, Spodoptera frugiperda “fall armyworm” was controlled by applying alpha cypermethrin and chlorpyrifos (800 mL/ha) plus Imidacloprid (700 g/ha). In addition, Diatraea saccharalis “stem borer” and Dalbulus maidis “corn leafhopper” were controlled by applying Emamectin Benzoate (100 g/ha) plus Imidacloprid (800 mL/ha). Additionally, Rhopalosiphum maidis “corn aphid” was controlled by applying Thiamethoxam (500 g/ha) and S. frugiperda “fall armyworm” by applying Emamectin Benzoate (100 g/ha) plus Imidacloprid (700 g/ha).
2.3.5. Fertilization of cowpea and corn crops
In each crop, two foliar fertilizations with biofertilizer were carried out at the dose of 2, 2.5 and 3 L/ha for treatments T3, T4, T5 respectively at 25 and 42 days after sowing. Moreover, two chemical fertilizations with commercial urea were carried out at a dose of 356 kg/ha for treatments T2 and T6 at 30 and 48 days after planting. In addition, foliar fertilization was applied to the last treatment (T6) at a dose of 2.5 L/ha.
2.3.6. Harvesting of cowpea and corn crops
The manual harvest was carried out when 95% of the cowpea plants presented the pods in a dry state, and 87 days after the corn planting. Randomly, in the two central rows, plants were extracted from five strokes per row (two plants per stroke), totaling 20 plants per plot and 60 per treatment. The cowpea plants were left to dry for 72 hours to facilitate natural drying to subsequently shell and weigh the grain obtained. On the other hand, immediately after the harvest, the growth characteristics of the corn plants were determined, and the fresh biomass was weighed, which was left to dry in a shaded environment to record the weight of the dry biomass after 14 days.
2.3.7. Assessments of cowpea and corn crops
Plant height, root length, number of pods per plant, number of grains per pod, weight of 100 seeds, and grain yield per treatment (20 plants per plot) were recorded for the 60 cowpea plants. To determine the number of grains per pod, 20 mature pods were randomly selected among the 20 plants in each plot. To record the weight of 100 seeds, 100 grains were randomly selected (12% - 14% moisture) and the yield was calculated by weighing the grain of all the pods of the 20 plants per plot, expressing them in kg/m2.
Height, number of leaves, root length, fresh and dry root biomass, and fresh and dry aerial biomass per treatment (20 plants per plot) were recorded in the 60 corn plants. The yield was calculated with the weight of the fresh aerial biomass of the 20 plants per plot and expressed in kg/m2.
2.4. Effect of biofertilizer on the population of nitrogen-fixing rhizospheric microorganisms and inorganic phosphate solubilizers
The rhizospheric soil of the 60 plants selected for each treatment to determine crop yield was mixed and homogenized using the quartering and piling method (Contreras & Carreño, 2018), and later a representative sample of 0.1 kg was collected for microbiological and physicochemical analysis of the experimental soil.
2.5. Data processing and analysis
The parametric values of crop growth and yield were analyzed to establish their normality (Kolmogorov-Smirnov test), homoscedasticity (Levene's test), determine the differences between treatments (Analysis of Variance) and significance between them (Duncan's Multiple Range Test), all with a significance level of 0.05. With the non-parametric values (number of pods per plant and grains per pod of cowpea crops, as well as the number of leaves of corn crops), the Kruskall-Wallis and Games-Howell test were performed, with a significance level of 0.05. The program used for these analyzes was IBM SPSS Statistics version 28.
3. Results and discussion
3.1. Physicochemical and biological characteristics of the biofertilizer from fish wastes
The biofertilizer presented nitrogen (1.65%), phosphorus (2.98%) and potassium (1.25%), as well as calcium, magnesium, sodium, carbonates, bicarbonates, chlorides and sulfates. Regarding the microbiological characteristics, the biol presented < 1.8 NMP/100 mL of total coliforms, thermotolerants, E. coli and Salmonella sp. (Table 1), results similar to those obtained with various liquid fertilizers obtained with fish wastes reported by Choi (2020), Thendral & Geetha (2019), Gutiérrez et al. (2019), Delgado et al. (2019), and Rishitha & Rao (2019).
Table 1 Physicochemical* and microbiological characteristics of the biofertilizer from fish wastes
Physicochemical characteristics | Units | Values |
---|---|---|
pH | - | 4.30 |
Electrical conductivity | dS/m | 19.25 |
Humidity | % | 11.48 |
Dry material | % | 88.52 |
Ashes | % | 9.88 |
Organic material | % | 40.70 |
Carbon (C) | % | 23.60 |
Nitrogen (N) | % | 1.65 |
Phosphorus (P2O5) | % | 2.98 |
Potassium (K2O) | % | 1.25 |
C/N Ratio | - | 14.30 |
Calcium (CaO) | mg/L | 16800 |
Magnesium (MgO) | mg/L | 5300 |
Sodium (Na) | mg/L | 12029 |
Carbonates (CO3) | mg/L | NF** |
Bicarbonates (HCO3) | mg/L | 41175 |
Chlorides (Cl) | mg/L | 54315 |
Sulfates (SO4) | mg/L | 13128 |
Microbiological characteristics | Values (MPN 100 m/L) | |
Total coliforms | < 1.8 | |
Thermotolerant coliforms | < 1.8 | |
Escherichia coli | < 1.8 | |
Salmonella sp. | < 1.8 |
* Soil and Water Laboratory (LABSAF) of the National Institute of Agrarian Innovation (INIA). ** NF: No found.
Thendral & Geetha (2019) concluded that pH variations are indicators of the fermentative process. The initial pH value of 6.98 decreased to 4.15 after 4 days of fermentation, as an indicator of the stabilization of organic residues by the action of enzymes and microbial acidity. Fish wastes are rich in organic matter that should not be directly applied to the soil because its decomposition decreases oxygen and increases temperature, and the carbon dioxide and fatty acids formed by decomposition could immobilize nutrients. Therefore, organic matter must be stabilized in fermentative processes such as the one used to obtain biofertilizers from fish wastes.
Fish wastes contain 11.7% - 13.0% N; 2.2% - 16.2% P; 10.5% - 13.2% K and 7.2% - 21.0% Ca. Fish bones are made up of 60% - 70% minerals, mostly Ca and P. Fish scales are rich in nitrogen, as well as phosphorus and calcium (Ahuja et al., 2020).
Organic fertilizers are defined as organic residues that once decomposed, under controlled conditions, contain the necessary nutrients for the development of plants and improvement of the physical, chemical and biological characteristics of the soil. Under this definition, biofertilizer is an organic fertilizer from the anaerobic decomposition of fish wastes alone (Thendral & Geetha, 2019; Rishitha & Rao, 2019) or together with other residues or substances such as fresh manure, whey, bone meal, blood (Gutiérrez et al., 2019), eggshells (Herawati et al., 2020), effective microorganisms (Choi, 2020), yeasts and sugar (Delgado et al., 2019).
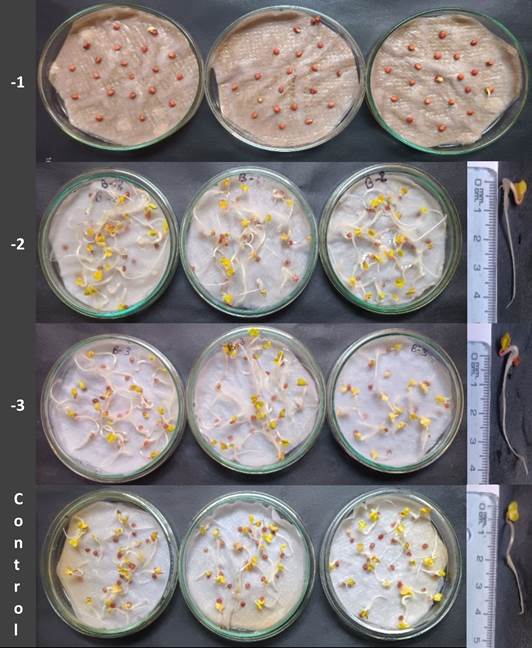
Figure 1 Seeds of Raphanus sativus L. "radish" germinated by the effect of applying three dilutions of biofertilizer from fish wastes.
Regarding the MPN of microorganisms in the biofertilizer, the values found coincide with those of Thendral & Geetha (2019), who recorded 0.3 MPN m/L of total coliforms and did not detect thermotolerant coliforms in the biofertilizer from fish wastes after 15 days of process. These researchers also quantified lactic acid bacteria (4.41 CFU m/L) and other bacteria, yeasts, and molds responsible for the acidity generated and decrease in pH that inhibits the growth of pathogens such as Salmonella sp. and Clostridium botulinum.
About the phytotoxicity, it was severe in the radish seeds in the 10-1 dilution and low in the 10-2 and 10-3 dilutions (Figure 1), while in the red onion seed-bulbs, the inhibition of root growth was 100% (10-1 dilution); 74.9% (10-2 dilution) and 13.1% (10-3 dilution) with respect to the first control, as well as 100% (10-1 dilution); 71.9% (10-2 dilution) and 2.5% (10-3 dilution) with respect to the second control.
The negative effect on germination is attributed to the fatty acids and ammonium present in the biofertilizer as a result of the fermentative process. Similarly, Thendral & Geetha (2019) reported that the germination rate of Vigna radiata seeds decreased to 75% with the 1:25 dilution and 50% with the 1:50 dilution of biofertilizer from fish wastes; however, a germination rate close to 150% was reached with the 1:100 dilution. Similarly, biofertilizer increased the germination rate of radish seeds to 178% (10-2) and decreased the growth inhibition of onion roots to 13% (10-3). According to Thendral & Geetha (2019), the decrease in phytotoxicity as dilution increases demonstrates that products such as biofertilizer from fish wastes can be used as agricultural fertilizers, and also, when the germination index is greater than 125%, they should be considered as biostimulants.
3.2. Effect of the application of chemical fertilizer and biofertilizer on Vigna unguiculata and Zea mays L.
The application of the three doses of biofertilizer (1%, 1.25% and 1.5%) increased plant height and root length, as well as the components and yield of the cowpea crop, compared to the absolute control. However, the increase percentages achieved with the biofertilizer were lower than those of the chemical fertilizer, which in turn was surpassed by the biol 1.25% plus chemical fertilizer (Figure 2).
The analysis of variance of the values of the cowpea parameters (Table 2) showed significant differences corresponding to plant height, root length, number of pods per plant, number of grains per pod, weight of 100 seeds and yield in grain. Duncan's Multiple Range test showed that the highest height, root length, weight of 100 seeds and grain yield was achieved with the application of biofertilizer at 1.25% plus chemical fertilizer. Regarding grain yield, a value of 0.48 kg/m2 was reached when applying biofertilizer at 1.25%, an identical value to that obtained with the chemical control (0.61 kg/m2).
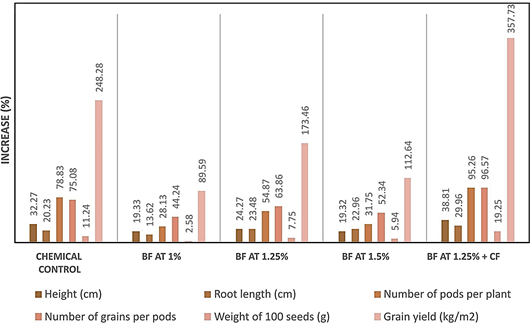
Figure 2 Percentage increase of growth parameters and yield components of Vigna unguiculata L. Walp crops due to the application of chemical fertilizer and three doses of biofertilizer. BF: Biofertilizer; CF: Chemical fertilizer.
Table 2 Growth parameters and yield components of Vigna unguiculata L. Walp. by effect of the application of chemical fertilizer and three doses of biofertilizer
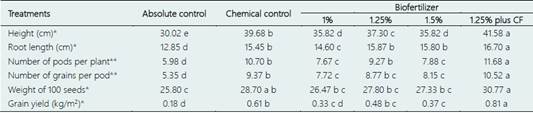
Different letters denote statistical significance. CF: Chemical fertilizer. *Duncan's Multiple Range Test (p < 0.05).
**Games - Howell test (p < 0.05).
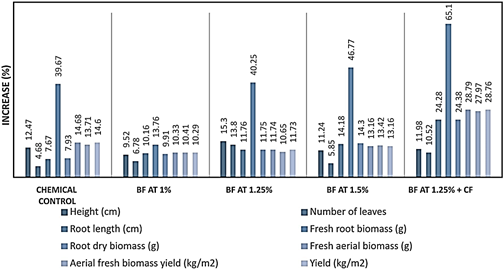
Figure 3 Percentage increase of the growth parameters and components of the crop yield of Zea mays L. due to the effect of the application of chemical fertilizer and three doses of biofertilizer. BF: Biofertilizer; CF: Chemical fertilizer.
Table 3 Growth parameters and yield components of Zea mays L. by effect of the application of chemical fertilizer and three doses of biofertilizer
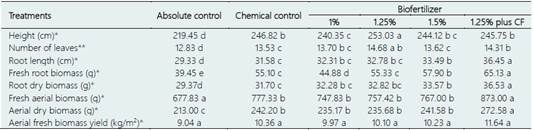
Different letters denote statistical significance. CF: Chemical fertilizer. *Duncan's Multiple Range Test (p < 0.05).
**Games - Howell test (p < 0.05).
The application of three doses of biofertilizer (1%, 1.25% and 1.5%) increased the parameters of vegetative growth and the yield in fresh and dry aerial biomass of corn, in comparison with the absolute control. The percentages of increase achieved with the biofertilizer exceeded the chemical control in terms of the number of leaves, root length, fresh and dry root biomass, but not in the fresh and dry aerial biomass or in the fresh aerial biomass yield, in which increases of 13.16%, 13.42% and 13.16% (biofertilizer) and 14.68%, 13.71% and 14.60% (chemical control) were reached, respectively. The percentages of increase achieved with the bio-fertilizer at 1.25% plus chemical fertilizer exceeded the biofertilizer at 1%, 1.25%, 1.5%, and the chemical fertilizer (Figure 3).
The analysis of variance of the values of the corn parameters (Table 3) showed significant differences corresponding to plant height, root length, fresh and dry root biomass, and fresh and dry aerial biomass, but not in aerial biomass fresh yield. Duncan's Multiple Range test showed that the highest values in root length, fresh and dry root biomass, fresh and dry aerial biomass, and fresh aerial biomass yield were reached with biofertilizer at 1.25% plus chemical fertilizer. Regarding the fresh and dry aerial biomass, the values corresponding to fertilizer at 1%, 1.25% and 1.5% were statistically equal to the chemical control.
The promotion of growth and yield of agricultural crops by liquid fertilizers from fish wastes has been previously reported in several crops. For example, in 30-day-old Pennisetum galucum and Trigonella foenum-graecum plants, an increase in the length of the leaves, stem and roots was demonstrated due to the application of liquid fertilizers from Catla catla (T1), Labeo rohita residues (T2) and a mixture of T1 and T2 (T3) fermented with Bacillus flexus and Pseudomonas stutzeri for 4 days (Rishitha & Rao, 2019); in 30-day-old tomato plants, an increase in height and number of leaves was demonstrated (Aranganathan & Radhika, 2016); in 56-day-old soybean plants, the plant height increased (Herawati et al., 2020); in alfalfa, plant height and biomass of leaves and stems increased (Gutiérrez et al., 2019); and in lettuce, the number and green color of the leaves was enhanced (Delgado et al., 2019).
Table 4 Population of rhizospheric microorganisms in cowpea and corn by effect of the application of chemical fertilizer and biofertilizer
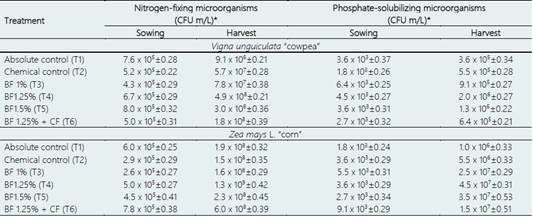
BF: Biofertilizer. CF: Chemical fertilizer. *Three repetitions.
Food products obtained from organic agriculture have a higher level of vitamin C, iron, magnesium, phosphorus and antioxidants, in addition to a better flavor and nutritional quality, and a minimum con tent of nitrate and pesticide residues; however, non-polluting agriculture has the disadvantage of lower yield than that obtained with conventional agriculture. Inorganic fertilizers have a greater amount of nutrients than organic ones, and also, they are immediately available to the plant, a con dition that result in a higher yield (Timsina, 2018). In this context, the superiority of the increase in yield obtained in cowpea with the chemical control (248.28%) and biofertilizer plus chemical fertilizer (357.73%) compared to biofertilizer at different doses (89.59% - 173.46%) is explained; as well as the increase observed in corn with the chemical control (14.6%) and biofertilizer plus chemical fertilizer (28.76%) compared to biofertilizer at different doses (29% - 13.16%).
3.3. Effect of the application of chemical fertilizer and biofertilizer on the microbial fertility of the soil
The application of the three doses of biofertilizer from fish wastes increased the microbial fertility of the soil, expressed as nitrogen-fixing and phosphate-solubilizing microorganisms per gram of soil. At the cowpea harvest, the application of three doses of biofertilizer increased the number of CFUs of nitrogen-fixing and phosphate-solubilizing microorganisms per gram of soil, compared to the absolute and chemical controls. Likewise, the application of biofertilizer at 1.25% surpassed the application of biol at 1.25% plus chemical fertilizer. On the other hand, in the case of corn harvest, the number of CFUs of nitrogen-fixing and phosphate-solubilizing microorganisms achieved with the application of the three doses of biofertilizer increased compared to the absolute control, chemical control, and the application of biofertilizer at 1.25% plus chemical fertilizer (Table 4).
The increase in nitrogen-fixing and phosphate-solubilizing microorganisms in the soil cultivated with cowpea and corn, due to the application of biofertilizer compared to the chemical control, evidenced the capacity of liquid fertilizer to improve soil fertility. This coincides with Choi (2020), who determined a significant increase in the length and biomass of earthworms, number of invertebrates and operational taxonomic bacterial units (OTU) in the soil cultivated with tomato. in which a liquid fertilizer from fish wastes was applied twice a week, during a period of 120 days.
The number of nitrogen-fixing and phosphate-solubilizing microorganisms was higher in the soil where biofertilizer at 1.25% plus chemical fertilizer (T6) was applied compared to the chemical control (T2), an apparently contradictory result that can be explained by the beneficial effect of organic matter from fish wastes. In the soil, the excessive application of chemical fertilizer changes its structure and acidifies it (Timsina, 2018), reducing the level of secondary macronutrients such as calcium, magnesium and sulfur, increasing heavy metals such as lead (Ugulu et al., 2020), and reduce the microbial diversity of the soil that actively participates in biogeochemical cycles and in nutrient recycling (Wei et al., 2017); however, when applied together with organic matter, the negative impact of chemical products decreases. In this regard, Ding et al. (2016) deter mined that a chemical fertilizer of nitrogen, phos phorus and potassium (NPK) increased the number of fungi, especially Fusarium and Gibberella genera, but their population was minimal when chemical fertilizer plus horse manure was applied. On the other hand, the population of beneficial fungi was higher in NPK plus manure, and much lower applying NPK.
The organic matter of the biofertilizer decreased the negative impact of chemical fertilizer on the soil, which is corroborated by Ning et al. (2017), who determined the effect of applying chemical fertilizer at 100% (T1), 80% (T2), 60% plus 20% of organic matter (T3), and 40% plus 40% of organic matter (T4) on soil fertility and microbial enzymatic activity. This research demonstrated that the reduction of chemical fertilizer did not have a significant effect on the amount of organic matter and catalase activity in the soil, reduced nitrogen, phosphorus and potassium and urease activity, and decreased phosphatase activity. On the other hand, the application of the chemical fertilizer with organic matter (T3 and T4) increased organic matter, as well as the microbial catalase and urease activity.
Furthermore, the six macronutrients N, P, K, Ca, Mg and S make up a significant part of the 30 grams of minerals that each kilogram of fresh plant material contains. Fertile soils provide a significant part of these elements; however, most crops depend on the external application of chemical fertilizers to satisfy the nutritional requirement. In this context, fertilizers based on fish wastes such as biofertilizer, in addition to having N, P, K, Ca, Mg and S, have organic matter, vitamins, growth regulators and beneficial microorganisms that stimulate the growth and yield of plants, as well as soil fertility (Ahuja et al., 2020).
4. Conclusions
The application of three doses of biofertilizer from fish wastes (1%, 1.25% and 1.5%) increased the vegetative growth parameters and the yield of the cowpea and corn crops compared to the absolute control. The best observed treatment was biofertilizer at 1.25% plus chemical fertilizer in the evaluated crops. In addition, the biofertilizer increased the microbial fertility of the soil expressed as the number of colony-forming units of nitrogen-fixing and phosphate-solubilizing microorganisms per gram of soil at harvest of cowpea and corn compared to the absolute and chemical controls. The results of this research validate the possibility of reducing the dose of chemical fertilizer (50%) in crops of economic importance as part of sustainable agricultural management. Finally, this study opens the way to investigate the effect on agricultural growth and yield when the biofertilizer from fish waste and plant growth-promoting microorganisms are jointly applied to crops of economic importance.