INTRODUCTION
Ovaries from mammalian species such as bovines contain thousands of immature oocytes enclosed in preantral follicles (about 90 to 95%), representing a potential source of fertilizable gametes (Mclaughlin et al., 2010). Because almost all oocytes (99.9%) suffer atresia if they remain in the ovaries (Figueiredo et al., 2008), it would be adequate to improve the procedure for preserving preantral follicles (PFs).
Cooling decreases the oocyte´s metabolism, minimizing energy use and slowing down the degeneration process (Roy and Treacy, 1993; Celestino et al., 2008; Chaves et al., 2008; Luna and Munhoz, 2008). This step is essential for preserving the viability of PFs during the transport of ovaries or preparation of routine procedures in the laboratory (cryopreservation protocols or in vitro follicle culture, or both).
Vitrification is an essential tool for the preservation of follicular morphology and viability over long periods. This process uses high concentrations of cryoprotective agents providing viscosity to the vitrification solution (Chian et al., 2004), avoids the damaging effects of ice crystal formation (Acker et al., 2001), and is a quick and straightforward procedure (Santos et al., 2007; Huang et al., 2008; Keros et al., 2009). However, the current cryopreservation process involves different steps and might cause damages to the cell structure (cell membrane, cytoplasmic organelles, and cell nucleus), as well as to DNA and the metabolism by the formation of reactive oxygen species (ROS) (Frei et al., 1989; Carvalho et al., 2013). The accumulation of ROS in the cell promotes oxidative stress, characterized by a disturbance between prooxidant and antioxidant systems of the cell (Agarwal et al., 2005).
Protection against ROS can be provided by enzymatic degradation, removal by antioxidants, and molecular repair. The addition of antioxidant agents such as ascorbic acid (AA), a non-enzymatic, water-soluble vitamin, has been investigated in different processes and species. It is involved in apoptosis processes due to its ability to protect cells from free radicals (Luck et al., 1995). Granulosa, theca, and luteal cells, oocytes, and follicular fluid are exceptionally rich in AA (Luck et al., 1995). Studies report the efficient effects of AA on embryo cryopreservation in mouse (Lane et al., 2002), ovine (Melo et al., 2011), bovine (Korhonen et al., 2012), and porcine (Castillo-Martin et al., 2013, 2014a, 2014b), as well as in the in vitro culture of PFs in mouse (Murray et al., 2001), bovine (Thomas et al., 2001; Andrade et al., 2012), caprine (Rossetto et al., 2009; Silva et al., 2011), and equine (Gomes et al., 2015).
Accordingly, the aim of this study was to evaluate the influence of adding AA to the vitrification medium on the morphology and viability of bovine PFs subjected to previous cooling at 4 °C during 4 or 24 h in TCM-199 medium.
MATERIALS AND METHODS
Ethical Considerations
All experimental procedures were approved by the Ethics Commission on the Use of Production Animals of Universidade Federal de Viçosa, Brazil [Process N.° 41/ 2014].
Source of Chemicals and Ovaries
Unless otherwise specified, the vitrification media and other chemicals used in the current study were purchased from Sigma Chemical Co. (Sigma, St Louis, USA). Ovaries (n=20) were collected at a local slaughterhouse from 10 Nelore heifers, 1416 months old. Ovaries were washed in 70% ethanol and then twice in transport medium, which consisted of tissue culture medium 199 (TCM-199) supplemented with 4-(2hydroxyethyl)-1-piperazineethanesulfonic acid (HEPES), penicillin (100 ìg/ml), and streptomycin (100 ìg/ml), and immediately transported to the laboratory at 4 ºC.
Experimental Design
Ten ovaries were used to assess the morphology of follicles, and 22 fragments (approximately 9 mm³) were removed from the cortical region of each ovary and distributed as follows: two fragments were used as fresh control (C0h), and 20 fragments were cooled at 4 ºC for periods of 4 and 24 h in TCM-199 plus HEPES and antibiotics (Figure 1). Of the 20 cooled fragments, two were fixed as controls for each cooling time (C4h, C24h) and the remaining 16 fragments were distributed in four vitrification treatments as indicated in Table 1.
Table 1 Vitrification treatments of bovine preantral follicles

V, Vitrification solutions; TCM-199, Tissue Culture Medium-199; DMSO, dimethyl sulfoxide; EG, ethylene glycol; SUC, sucrose; AA, ascorbic acid
After vitrification, the fragments were placed in cryotubes and stored in liquid nitrogen for 72 h. Subsequently, they were warmed and the cryoprotectants were removed in decreasing solutions of SUC (0.5, 0.25, and 0 M). The tissue pieces were then directly fixed for morphology analysis. The other bovine ovaries (n= 10) were fragmented and distributed as described above to evaluate the viability of PFs. Then, fragments were subjected to mechanical follicular isolation for viability analysis.
Morphological Analysis of Bovine PFs
Fragments from the fresh control (C0h), cooled controls (C4h and C24h), and the vitrified ones after warming and removal of the cryoprotectant were fixed in Carnoy's solution during 4 h for morphological analysis by classic histology. Samples were dehydrated in increasing alcohol concentrations (70, 80, and 90%) and then in three baths of absolute alcohol, remaining 1 h in each. Subsequently, they were diaphanized in three baths of xylene and embedded into paraffin to produce serials with thicknesses of 5 µm. Every 6th section was mounted on slides and stained through the Periodic Acid-Schiff (PAS)-Hematoxylin method (Carvalho et al., 2011). Slides were examined under an optical microscope (Olympus BX60, Japan) at 400X magnification, and 30 preantral follicles per slide with five replicates were used (450 in total and 150 PFs per treatment).
According to the developmental stage, the preantral follicles were classified as 1) primordial (one layer of flattened granulosa cells around the oocyte); 2) transition (one layer of flattened to cuboidal granulosa cells); 3) primary (one layer of cubic granulosa cells); and 4) secondary (two or more layers of cuboidal granulosa cells around the oocyte) (Figueiredo et al., 2008). These follicles were classified individually as morphologically normal when an intact oocyte was present and surrounded by well-organized granulosa cells in one or more layers without a pyknotic nuclei.Atretic follicles were defined as those with a retracted oocyte, pyknotic nucleus, or disorganized granulosa cells detached from the basement membrane, or both.
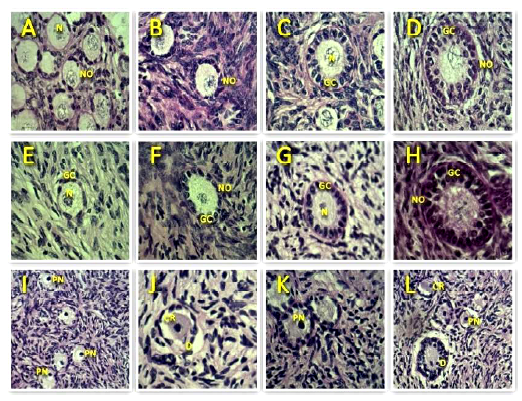
Figure 2 Representative images of the morphology of bovine preantral follicles after staining with periodic acid Schiff-hematoxylin. Normal follicles are shown in control (A, B, C, D) and after vitrification (E, F, G, H), while degenerate follicles are represented in I, J, K, L. N-nucleolus; NO-normal oocyte; PN-pyknotic oocyte nucleus; CR-cytoplasmic retraction; GC-granulosa cells; D-disorganized granulosa cells (400x). Scale bars = 50 μm
Viability Analysis of Bovine PFs
Bovine PFs were isolated from ovarian tissue using a mechanical procedure. Fragments from all treatments were finely cut with a scalpel blade in longitudinal, transverse, and oblique axes, from the outer to the inside region of the ovarian cortex; then, these fragments were placed in TCM-199 medium added with 10% Bovine Serum Albumin. The fragments were suspended by repeated pipetting movements (100 times) with a large Pasteur pipette (1000 µm in diameter) and subsequently, with a smaller pipette of 600 µm in diameter (100 times) to release the preantral follicles. The suspension was filtered twice through a 100 µm nylon mesh. Then, PF viability was assessed by Trypan Blue staining at 0.4%, adding 5 µl on each 100 µl of PF isolate solution, and incubated for 1 min at room temperature. Next, the PFs were examined to assess the cell membrane integrity through dye penetration (Amorim et al., 2003) using an inverted microscope (Olympus, Japan). PFs were rated as dead or alive when stained or unstained with Trypan Blue, respectively. In total, 150 follicles per treatment were analyzed, totalizing 1650 follicles with five replicates per treatment.
Statistical Analysis
Data were initially subjected to Shapiro-Wilk and Bartlett's tests to confirm normal distribution and homogeneity of variance, respectively.An analysis of variance was then carried out using the GLM procedure (SAS, 2002). The comparison of means among treatment was performed using the F test. Results were expressed as mean ± standard error of the mean. The significance level adopted was α = 0.05.
The experiment was carried out in a factorial design plus three additional treatments (4 x 2 + 3): four vitrification solutions (V, VSUC, VAA, and VSUC+AA), two cooling times (4 h and 24 h), and three controls (C0h, C4h, and C24h). This mathematical model was the following: Yijk+= µ + ti + vj + ti*vj + eijk, and yk = µ + á + e, where y = response variable; µ = constant; ti = cooling time effect (4h, 24 h); vj = vitrification solution effect(V, VSUC, VAA, VSUC+AA); ti*vj = interaction of cooling time and vitrification solution; a = additional treatment effect, and e = error.
RESULTS
Morphological analysis of bovine PFs after cooling and vitrification
PFs in situ were evaluated and classified according to the development stage and qualified morphologically as normal or degenerated (Table 2). A high presence of primordial follicles (49%; 808/1650) was recorded. Morphologically normal bovine PFs according to the developmental stage (primordial, transition, primary and secondary) were observed in control groups and after vitrification (Figure 1).
Table 2 Classification and qualification of preantral follicles (PFs) included in bovine ovarian tissue
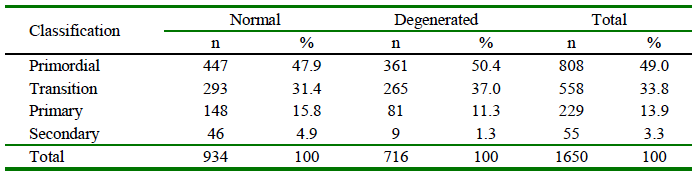
The percentage of morphologically normal follicles did not differ between the fresh control (C0h), and the C4h cooled group (p>0.05); nevertheless, the latter showed a significant decrease (p<0.01) in the percentage of normal PFs compared to C0h (Table 3).
Table 3 Morphologically normal and viable preantral follicles (PFs) according to the cooling time before and after vitrification (n=150 per treatment)
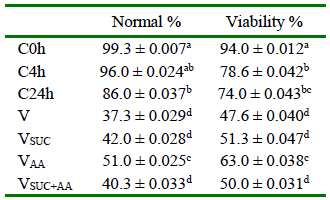
a,b,c,d superscript lowercase letters in the same column are different (p<0.05).
C0h, fresh control; C4h, Preantral follicles (PFs) cooled at 4 °C for 4 h without further vitrification; C24h, PFs cooled at 4 °C for 24 h without further vitrification; V, Vitrification solutions with TCM199 + (DMSO) dimethyl sulfoxide, and (EG) ethylene glycol; VSUC, Vitrification solutions with TCM-199 + DMSO + EG, and (SUC) sucrose; VAA, Vitrification solutions with TCM-199 + DMSO + EG, and (AA) ascorbic acid; VSUC+AA, Vitrification solutions with TCM-199 + DMSO + EG + SUC, and AA
No interaction between cooling time and vitrification treatments was detected (p>0.05). After PF vitrification and warming, the morphology and viability in all vitrification treatments were inferior to C0h (p<0.05). However, the VAA treatment showed greater normal follicles than the other vitrification treatments (Table 3), with a high number of follicles with intact nucleolus and normal oocyte surrounded by well-arranged granulosa cells.
Viability analysis of bovine PFs after cooling and vitrification
There were no differences between cooling times in the viability analysis (p>0.05); however, both C4h and C24h treatments reduced the viability (78.7 and 74%, respectively) when compared to the fresh control (94%; p<0.01). The trypan blue viability analysis showed that only the VAA treatment maintained follicular viability similar to C24h (63 and 74%, respectively; p>0.05; Table 3).
DISCUSSION
The absence of vascular support during ovary transport to the laboratory impairs the supplementation of oxygen and energy to the ovaries (Chaves et al., 2008). Oxygen deprivation of the tissue results in a change from aerobic to anaerobic metabolism, with lactic acid being the main product accumulated within the cell, causing pH reduction and cell death (Wongsrikeao et al., 2005). These effects were reduced through cooling to induce a reduction in cellular metabolism, increasing follicle resistance to nutrient and oxygen reduction during in vitro preservation. The cooling of ovarian fragments at 4 °C allowed to maintain high percentages of normal PFs similar to the fresh control follicles for 4 h in TCM-199 medium. On this respect, Celestino et al. (2007) found that the PFs can be preserved efficiently at 4 ºC for up to 24 h in this same medium. However, in this study, ovarian fragments cooled for 24 h showed an increased percentage of degenerated PFs, probably due to alterations in cell membrane permeability induced by the lack of oxygen that, in turn, can lead to an increase in intracellular water and cell volume, cytoplasmic vacuolization, and consequently, cellular degeneration (Barros et al., 2001).
Good preservation of ovaries in TCM199 medium was also demonstrated in goats (Ferreira et al., 2001; Costa et al., 2005) and sheep (Matos et al., 2004). In the current study, bovine primordial follicles survived with their energy reserves and TCM-199 nutrients like glucose, inorganic salts, vitamins, and amino acids for 4 h before vitrification, indicating that primordial follicles are resistant to low temperatures and ischemia.
The preservation of PFs may be performed for a short (cooling) or a long (cryopreservation) period. The combination of these two techniques is essential to ensure that the cells remain at a low metabolic rate for a specified storage time to be retrieved and continue their in vitro development. Regardless of the cooling time, all PFs that were vitrified showed a reduction in the percentage of normal morphology. Injuries caused to the cells may be assigned to the intense release of ROS.According toAgarwal et al. (2005), the increase of ROS in the cell promotes oxidative stress characterized by a disturbance between oxidant and antioxidant cellular systems.
Both morphological and viability analyses showed that addition of AA could reduce cellular damage caused by the cryopreservation process. AA is a potent antioxidant that accumulates in granulosa, inner theca, luteal, and oocyte cells (Thomas et al., 2001). The AA mechanism of action is quite varied. Sagun et al. (2005) found that the oxidized form of AA, dehydroascorbic acid (DHA), enters the mitochondria via facilitative glucose transporter 1 (Glut1) and accumulates into mitochondria as ascorbic acid. Mitochondrial AA quenches ROS protects the mitochondrial genome, and inhibits mitochondrial membrane depolarization, inhibiting oxidative stress. In this way, the antioxidant properties ofAAare attributed to its capacity to reduce the damage from ROS (Buettner, 1993) and metal-oxygen complexes to DNA, proteins, carbohydrates, lipids, and cell membranes (Fraga et al., 1991; Sies et al., 1992; Luck et al., 1995). Additionally, AA plays a role in the biosynthesis of steroid and peptide hormones and the process of collagen synthesis (Luck et al., 1995), thus, being important during follicular growth, ovulation and corpus luteum formation (Li and Schellhorn, 2007).