1. Introduction
Pathogenic microorganisms are the main cause of hydrobiological food contamination (Walczak et al., 2017). In fish, these pathogens alter their quality and reduce fish shelf-life, making them not able for human consumption (Peña et al., 2019). Due to these pathogens, foodborne diseases also occur, which constitute a serious health problem worldwide (Soto et al., 2016).
Pathogens can cause alterations in the nutritional and organoleptic properties of foods (Rani et al., 2016); therefore, their detection is very important (Law et al., 2015). To solve this problem, synthetic antimicrobial agents are used although it is currently replaced by compounds from natural origin (Mostafa et al., 2018; Pastrana-Puche et al., 2017). There has been an increase in the search for efficient alternative for food preservation (Savoldi et al., 2020; Bachir & Benali, 2012); and different “in vitro” studies demonstrate the efficacy of spices to inhibit pathogenic microorganisms (Huong et al., 2021; El Jery et al., 2020).
There are many possible useful bioactive substances that are derived from plants (Bajpai et al., 2012). It is the case of essential oils which have antimicrobial properties. They are composed of a variety of active components such as: terpenes, terpenoids, carotenoids, coumarins and curcumin. (Calo et al., 2015; Cevallos & Londoño, 2017; Pandey et al., 2017). Due to the inhibitory activity that reduces the growth of bacteria, yeasts and fungi, essential oils are a promising alternative for food preservation (Mancuso et al., 2019).
Most essential oils are obtained by steam distillation and hydro-distillation; these techniques are widely used because of its high performance; the purity of the obtained essential oil and it does not require sophisticated technology (Patiño & Martínez, 2014). However, there are others extraction methods such as hydrodistillation assisted by microwave, solvents extraction, among others.
To determine the antimicrobial activity "in vitro" against different pathogenic microorganisms, different methods are used such as: macrodilution or tube dilution method, microdilution or plate dilution, disk diffusion or Kirby-Bauer method, among others which evaluate minimum inhibitory concentration (MIC) and minimum bactericidal concentration (MBC) (Laith, 2021; Parvekar et al., 2020; Wijesinghe et al., 2021).
Bursera graveolens it is a deciduous tree that grows in the lower part of the western slopes of northern Peru, and it is called “palo santo” in these zones (Puescas, 2010). It is an aromatic plant that is used for traditional medicine. However, the production of the essential oil of this species is attractive because of its applications (Preedy, 2015). Fon-Fay et al. (2017) analyzed "in vitro" antimicrobial activity of B. graveolens against Escherichia coli, Staphylococcus aureus, Bacillus subtilis, Salmonella enteritidis, Aspergillus niger and Penicillium citrinum; finding a MIC of 0.005 μl/ml for Staphylococcus aureus. Celina Luján-Hidalgo et al. (2012) studied the antimicrobial activity of the essential oil from B. graveolens leaves against S. aureus and B. subtilis (MIC of 7.45 mg/ml and 7.29 mg/ml, respectively). Additionally, the authors, found MIC of 1.71 mg/ml to 11.99 mg/ml for yeast. The antimicrobial activity of the essential oil of B. graveolens branches has also been investigated by Sotelo-Méndez et al. (2017). It was found that that the essential oil has significant antimicrobial properties against S. aureus, Bacillus cereus, Listeria monocytogenes, Clostridium perfringens, E. coli, Salmonella choleraesuis, and Candida albicans
Although the antimicrobial capacity of B. graveolens essential oil has been demonstrated in the previous studies, the origin (type of food) of the microbial strains used is not specified. These results might not be applicable for the most of kind of food. To stablish effective strategies for specific kind of food, the antimicrobial test must be done against microorganisms isolated from the same food. In the present study, the "in vitro" antimicrobial activity of Bursera graveolens essential oil was determined against microorganisms isolated and molecular identified from Kajikia audax.
2. Materials and methods
2.1 Essential oil extraction from B. graveolens
The extraction process was carried out by steam distillation. The raw material was obtained in the district of Rica Playa- Quebrada La Sesina (3-48'42"S 80-31'54"W), belonging to the national park Cerros de Amotape Tumbes - Peru which consisted of 3 pieces of the trunk of B. graveolens with weights of: 2.61 kg, 3.32 kg and 2.41 kg, of which with the help of an electric carpentry planer a total of 6.98 kg was obtained. The swarf was put in an autoclave machine and without coming in contact with the water, was subjected to a heat source for 1 h 45 minutes, the steam produced by the autoclave was circulated as a water coolant by a distiller. Once becoming an aqueous solution, with the help of a separatory funnel, the oil was separated from the water by difference of weights, thus obtaining 105 ml B. graveolens essential oil that was then stored in an amber bottle in refrigeration.
2.2 Yield determination
The yield of B. graveolens essential oil, was obtained in terms of percentage by weight (%) based on the mass of the dry material used (Kg), using the following formula (Cruz et al., 2013): /; where Y%: yield in %; Mass.EO: mass of essential oil; Mass.MV: mass of the material placed in extractor “g”.
2.3 Chemical characterization of the essential oil
Chemical characterization was performed by Agilent Technologies 7890A gas chromatograph with Agilent Technologies 5975C mass spectrophotometer detector, with the following chromatographic conditions: column J&W 122-1545.67659 DB-5ms, 325 °C: 60 m x 250 m x 0.25 mm. Thermal profiling: starting at 40 °C it rises 5 °C/min up to 180 °C; then 2.5 °C/min up to 200 °C where it stays for 5 min then finally rises 10 °C/min up to 300 °C for 3 min. The sample injected was 1 µl which was obtained from the mixture of 20 µl of essential oil in 1 ml of dichloromethane. The run time was 54 minutes, and the carrier gas was helium (1 ml/min).
2.4 Determination of density, refractive index and maximum absorbance
The density was obtained using a standardized pycnometer, which was weighed beforehand on an analytical balance for an accurate measure, then the weight of the essential oil sample was scaled. For the measurement of refractive index, a type of refractometer (ABBE Schmidt Haensh, Germany) was used. The method based on ISO 4735 was used to measure the maximum absorbance in the ultraviolet radiation range, which is based on the determination of the absorption spectrum of the alcoholic dissolution of the essential oil (250 mg in 100 ml ethyl alcohol), using pure ethanol, in the wavelength range between 260 and 340 nm, a spectrophotometer was used for these measurements (LAMBDA 365 double-beam UV/Vis Spectrophotometer by PerkinElmer, USA) (Cruz et al., 2013).
2.5 Isolation and identification of microorganisms
In a flask, a sample of 25 g of Kajikia audax was weighed, then at room temperature 225 ml of diluent (1% peptone water) was added. Successive dilutions were performed up to 10-3, and in petri dishes 0.1 ml of this dilution was inoculated with agar: Eosin methylene blue (EMB) agar or Endo agar for isolation of Escherichia coli and enterobacteria; Baird-Parker agar for staphylococci; Thiosulfate-citrate-bile salts-sucrose (TCBS) agar for vibrio; Salmonella Shigella (SS) agar for Salmonella and Sabouraud agar for fungi and yeast (DIGESA, 2008). To demonstrate cell morphology Gram staining was used and for the identification of each strain the PCR (Polymerase chain reaction) of the 16S rRNA gene was used for bacteria (Wang et al., 2011) and ITS (Internal transcribed spacer) for fungi (White et al., 1990) with prior sequencing by the company Macrogen Inc (Korea).
2.6 Determination of the minimum inhibitory concentration (MIC) and the minimum bactericidal concentration (MBC) by tube dilution method
A stock solution was prepared by adding 2 ml of B. graveolens essential oil, 2ml of DMSO (Dimethyl sulfoxide) and 12 ml of Mueller Hinton Broth (MHB) to a flask (Hili et al., 1997). The inoculum was prepared with microorganisms developed in Muller Hinton Broth for 18 to 24 hours, with a bacterial suspension adjusted to the McFarland standard of 0.5 and a 1/10 dilution getting a final concentration of 107 CFU/ml. From the stock solution, serial dilutions were made, preparing 10 test tubes with the following concentrations of essential oil: 207.33 mg/ml; 103.67 mg/ml; 58.3 mg/ml, 25.91 mg/ml; 12.96 mg/ml; 6.48 mg/ml; 3.24 mg/ml; 1.62 mg/ml; 0.81 mg/ml; 0.41 mg/ml. To each tube, 10 μl of the inoculum was added. Two test tubes were kept without being inoculated: a broth tube as a negative growth control and a tube with essential oil as a positive growth control. The concentration of essential oil that does not show growth is the minimum inhibitory concentration (MIC). With the help of an inoculation loop a sample from the tubes where no turbidity was observed was transferred to a plate with Mueller-Hinton agar. Thus, the content of the positive control tube should develop growth in the dish, and the concentration where no growth appears in the plate will correspond to the minimum bactericidal concentration (MBC) (Servicios Antimicrobianos, 2012).
3. Results and discussion
3.1 Yield and characterization of B. graveolens essential oil
Table 1 presents the organoleptic and physicochemical properties of the essential oil. The density and refractive index were close to those reported before in the essential oil from the leaves and fruits of B. graveolens (Cruz et al., 2013; Luján-Hidalgo et al., 2012; Rey-Valeirón et al., 2017). As for the yield, it was much higher than that found by Cruz et al. (2013) with a value of 0.09% and that found by Luján-Hidalgo et al. (2012) with a value of 0.10%. Consequently, it should be considered that certain circumstantial factors may have influenced the characteristics of the oil such the location from which the raw material was extracted (Leal-Torres et al., 2013), as well as the distillation time and the condition of the material (Meyer-Torres et al., 2018).
Table 1 Organoleptic and physicochemical properties of Bursera graveolens essential oil
Parameter | Observation |
Color | Clear light yellow |
Odor | Intense and pungent |
Taste | Spicy |
Density | 0.83 g/ml |
State | Liquid |
Yield | 1.25% |
Refractive Index | 1.473° |
Furthermore, the maximum absorption of the essential oil analyzed in Figure 1 paralleled with a wavelength (nm) close to 272.5 nm in the UV-VIS wavelength range, which was found below that reported by Cruz et al. (2013) at a value of 293 nm.
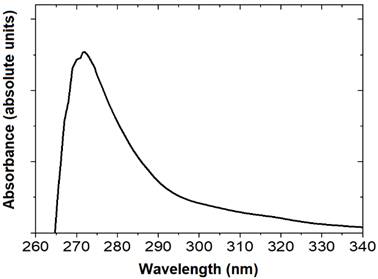
Figure 1 Absorption of Bursera graveolens essential oil obtained by Double-Beam UV-Vis Spectrophotometer.
The gas chromatography analysis of the extracted essen tial oil allowed the identification of 28 chemical com pounds making up 82.35% of the chemical composition of the essential oil, with 6 others being unknown. Table 2 shows the most important compounds, such as terpenes, monoterpenes, diterpenes, sesquiterpenes, and some oxygenated compounds found in this work in comparison with previous and recent works. The main constituents were menthofuran (5.16%), α-terpineol (4.01%), germacrene D (2.33%), carvone (0.83%). The largest compound was monoterpene D-limonene with 77.06%.
Studies related to the chemical composition of B. graveolens essential oil using the same method of analysis or a variation of it, mention monoterpenes as main constituents, namely limonene and menthofuran (Carmona et al., 2009; Monzote et al., 2012; Jaramillo-Colorado et al., 2019; Rey-Valeirón et al, 2017) or sesquiterpenes as cadinene, farnesene and germacrene-D (Luján-Hidalgo et al., 2012; Sotelo-Méndez et al., 2017). However, Sotelo-Méndez et al. (2017), Luján-Hidalgo et al. (2012), Rey-Valeirón et al. (2017) and Carmona et al. (2009) found other constituents of B. graveolens essential oil, which were not found with gas chromatography in the present study.
We may explain this difference by the source of where the oil was extracted, and some authors obtained the oil of the leaves (Luján-Hidalgo et al., 2012; Monzote et al., 2012) or fruits (Rey-Valeirón et al., 2017) or a mixture of stems and leaves (Jaramillo-Colorado et al., 2019) of B. graveolens, while in this research it was attained specifically from the part of the stem. Additionally, it may be due to some ecological conditions under which the plants persisted which may alter the qualitative and quantitative characteristics of the essential oil, such as those caused by the specific season the samples were gained (Sotelo-Méndez et al., 2017). In the chemical composition of the essential oil different terpenic compounds were found, such as monoterpenes and sesquiterpenes, that are aromatic compounds which contribute to the menthol aroma of the dry trunk of B. graveolens (Yukawa et al., 2006).
3.2 Isolation of microorganisms
From the sample of Kajikia audax, 6 strains were isolated out of which 2 were not viable. The identified microorganisms were: Aeromonas salmonicida (MK1), Pseudomonas aeruginosa (PS1), P. aeruginosa (PS2) and Pichia kudriavzevii (lev). The molecular identification is shown in Table 3, such as percent of similarity and the accession number of the organism type.
The microscopic characteristics of bacteria closely resemble Gram-negative bacteria and that of fungi to yeast (Figure 2).
Fish and shellfish have a diverse microbiota, which on some occasions belong to altering microorganisms and on others, to microorganisms that cause food-borne illnesses (Karthiga Rani et al., 2016). Among the pathogenic and spoilage bacteria in fish are the gram-negative rods of the families Enterobacteriaceae and Vibrionaceae, the Flavobacterium genus, the Pseudomonas genus and the Aeromonas genus. However, the species of these bacterial groups can be isolated from fresh fish, without causing appreciable disease (Walczak et al., 2017).
Within the Aeromonas genus, A. salmonicida is the etiological agent of furunculosis in salmonids, however, it is also isolated in non-salmonid fish (Sudheesh et al., 2012) such as Kajikia audax. Although its pathogenicity is referred to fish, its presence in food is associated to food spoiling and outbreak food contamination in humans (Pękala-Safińska et al., 2021).
Pseudomonas species are usually isolated in a significant percentage (63%) in fish samples, and it is considered as a microbiological quality indicator microorganism in food (Karthiga Rani et al., 2016). The presence of the genus Pseudomonas and the Shewanella genus has been observed both in the initial and final microbiota of the shelf-life fish, measured by 16 days (Parlapani et al., 2015). Therefore, its presence in isolates from fresh fish samples is frequent, as in the case of this study.
The presence of Pichia kudriavzevii (syn. Candida krusei syn. Issatchenkia orientalis) is associated to the microbiota of plants and soil. However, it has been suggested that the ecology of pathogenic yeasts is diverse, so it is possible that this yeast could be found in additional environments (Opulente et al., 2019), as in the case of fish species.
Table 3 Molecular bacterial identification of the strains isolated from Kajikia audax (Nucleotide BLAST)
Strain | Sequence size | Closer identification | % Similarity | Access number |
MK1 | 1418 | Aeromonas salmonicida | 99.86% | CP048223.1 |
PS1 | 1375 | Pseudomonas aeruginosa | 100.0% | CP053028.1 |
PS2 | 1388 | Pseudomonas aeruginosa | 100.0% | CP053028.1 |
Lev | 505 | Pichia kudriavzevii | 98.58% | LC389009 |
Although the microbiota of fish is diverse, also considering the species of microorganisms that arrive by handling the product, it should be considered that the different temperatures and atmospheres that are applied can affect the composition and growth of the microorganisms considered as altering microbiota (Parlapani et al., 2015).
3.3 Minimum Inhibitory Concentration (MIC) and Minimum Bactericidal Concentration (MBC)
The concentrations used were ranging from 0.41 mg/ml to 207.33 mg/ml. Table 4 shows the results of “in vitro” antimicrobial activity of B. graveolens essential oil as the MIC and MBC for each microorganism. Similarly, MBC plates can be observed on Figure 3.
Table 4 Minimum Inhibitory Concentration (MIC) and Minimum Bactericidal Concentration (MBC) of the essential oil against the microorganisms isolated from Kajikia audax
Microorganism | MIC (mg/ml) | MBC (mg/ml) |
Aeromonas salmonicida | 1.62 | 25.92 |
Pseudomonas aeruginosa | >207.33* | >207.33* |
Pseudomonas aeruginosa | >207.33* | >207.33* |
Pichia kudriavzevii | 6.48 | 25.92 |
*Inhibition above the concentration of 207.33 mg/ml was not tested.
Luján-Hidalgo et al. (2012) evaluated the “in vitro” antimicrobial activity of B. graveolens over E. coli and Citrobacter freundii and found that the essential oil did not show antimicrobial activity on these enterobacteria. This may prove that essential oils do not show the same antibacterial effect with strains of the same or different microbial family or class since A. salmonicida, of the Aeromonadaceae family, we determined an MIC of 1.6197 mg/ml and an MBC of 25.9156 mg/ml and as for the representatives of the Pseudomonadaceae family they had shown resistance. It should also be noted that the Enterobacteriaceae, Pseudomonadaceae, as well as the Aeromonadaceae families belong to the Gamma-proteobacteria class of the Proteobacteria phylum.
Moreover, Luján-Hidalgo et al. (2012) found that for gram-positive bacteria such as S. aureus, the MBC was 7.45 mg/ml and 7.29 mg/ml for Bacillus subtilis. In this case the results show a more effective antimicrobial effect for other Gammaproteobacteria. On the other hand, Sotelo-Méndez et al. (2017) found that when using essential oil obtained from dry logs of B. graveolens, it demonstrated a 100% inhibition against gram-positive bacteria, gram-negative bacteria and yeasts, due to the purity of the oil. Furthermore, the bacteria were sensitive to chloram-phenicol, oxytetracycline and fluconazole.
In the present work, bacteria were also proved to be sensitive to amikacin, A. salmonicida with inhibition halo of 30 mm, P. aeruginosa (PS1) 25 mm and P. aeruginosa (PS2) 18 mm. B. graveolens had shown a strong “in vitro” antimicrobial activity over E. coli, S. aureus, B. subtilis, Salmonella enteritidis, Aspertillus niger and Penicillium citrinum which made it necessary to set a range from 0.005 to 5 μl/ml for MIC for such bacteria (Fon-Fay et al., 2017). Similarly, Canales-Martínez et al. (2017) found antimicrobial activity of Bursera morelensis essential oil against Streptococcus pneumoniae, Vibrio cholerae and E. coli with a MIC of 0.125 mg/ml and an MBC of 0.25 mg/ml. The oil had also inhibited the filamentous fungi. In the case of yeasts, the present study had found higher values of MIC and MBC to that mentioned previously (6.4789 mg/ml and 25.9156 mg/ml, respectively). The caused inhibition in enterobacteria and yeast may be due to the high percentage of the monoterpene, D-limonene; and, to a lesser extent, the sesquiterpenes and diterpenes found in the chemical composition (Sotelo-Méndez et al., 2017; Luján-Hidalgo et al., 2012; Fernández-Ruiz et al., 2018). According to Loor & Coello (2019), D-limonene is an antioxidant metabolite of pharmaceutical interest, with anti-inflammatory, antibacterial and antifungal activity.
Researchers note that the antimicrobial activity of essential oils against the different pathogenic microorganisms present in food is due to its photochemical composition.
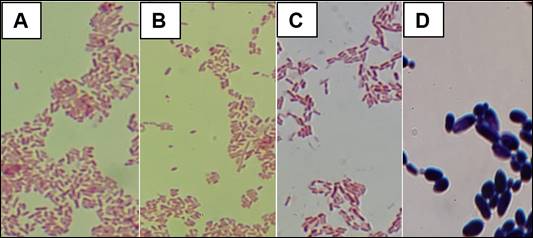
Figure 2 Gram stain and bacterial cell morphology. A : Aeromonas salmonicida, B and C: Pseudomonas aeruginosa and D: Pichia kudriavzevii (Direct microscopy, 1000x).
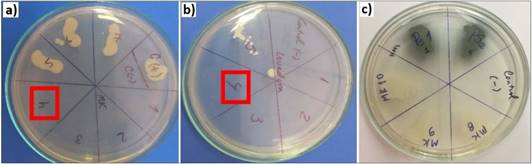
Figure 3 Minimum Bactericidal Concentration (red) of B. graveolens essencial oil over a) Aeromonas salmonicida (MK), b) Pichia kudriavzevii (Levadura), c) Pseudomonas aeruginosa (PS1 and PS2). Both P. aeruginosa strains presented MBC > 207.33 mg/ml, while the other strains presented MBC = 25.92 mg/ml.
Components such as terpenes, monoterpenes, sesquiterpenes, oxygenated and phenolic compounds are found to be responsible for a variety of biological activities (Pinto, 2014). To evaluate their antimicrobial activity, researchers had studied exclusively the specific chemical compounds present in the chemical composition of B. graveolens essential oil. Such is the case of the compound (+)-α-pinene which was dissolved in 1% Tween® 80 (otherwise known as Polysorbate 80) and in 5% DMSO (dimethyl sulfoxide) - that inhibited E. coli (ATCC® 25922™) - with a concentration of 160 μl/ml with an arithmetic mean of 12 mm of inhibition halo diameter (Farias et al., 2017).
Another compound find in essential oils is carvone, it has also been assessed "in vitro" against the bacterium, L. monocytogenes, attaining a MIC and MBC of 2.5 µL/ml, led to the conclusion that the bacteria are sensitive to this chemical component (Shahbazi, 2015). Lastly, the compound, α-terpineol has shown antifungal activity against Geotrichum citri-aurantii with a 2.00 μl/ml MIC and a minimum fungicidal concentration (MFC) of 4.00 μl/ml, causing an impairment in the integrity of the membrane of the cell and leakage of intracellular components (Zhou et al., 2014).
4. Conclusions
Chemical characterization of B. graveolens essential oil - attained by steam distillation- presented the following compounds as primary constituents identified by gas chromatography-mass spectrometry (GC-MS): D-limo-nene (77.06%), menthofuran (5.16%), α-terpineol (4.01%), germacrene D (2.33%) and carvone (0.83%). Furthermore, during the isolation of microorganisms of K. audax, gram-negative bacilli (A. salmonicida and P. aeruginosa) and a yeast (P. kudriavzevii) were distinguished. The minimum inhibitory concentration (MIC) and minimum bactericidal concentration (MBC) found for sensitive microorganisms showed a correlation to the antimicrobial properties present in the chemical composition of B. graveolens essential oil of, such as monoterpenes diterpenes, sesquiterpenes, oxygenated and phenolic compounds. This sensitivity makes it possible that B. graveolens essential oil can be utilized to control the growth of microorganisms in hydrobiological products. Neverthe-less, several environmental, chemical factors influencing toxicity as well as the susceptibility of other microorganisms in relation to the essential oil or its purified components must still be examined.