Services on Demand
Journal
Article
Indicators
-
Cited by SciELO
Related links
-
Similars in SciELO
Share
Industrial Data
Print version ISSN 1560-9146On-line version ISSN 1810-9993
Ind. data vol.25 no.2 Lima July/Dic. 2022
http://dx.doi.org/10.15381/idata.v25i2.23591
Design and Technology
Impact of Iron, Magnesium and Manganese on Arugula Chlorosis in Hydroponic Systems
5 Agroindustrial engineer from Universidad Nacional Federico Villarreal. Currently working as independent consultant (Lima, Perú). E-mail: 2017045465@unfv.edu.pe
2 PhD in Science and Biological Engineering from Universidad Nacional Agraria La Molina. Currently working as full professor at the Biology Department of the Science School of Universidad Nacional Agraria La Molina (Lima, Perú). E-mail: delfin@lamolina.edu.pe
3 PhD in Business Management from Universidad Nacional Mayor de San Marcos. Currently working as assistant professor at the Administration Department of the Administrative Sciences of Universidad Nacional Mayor de San Marcos and as analyst at Telefónica del Perú S.A.A (Lima, Perú). E-mail: wbazanr@unmsm.edu.pe
4 Degree in Human Nutrition from Universidad Norbert Wiener. Currently working as an independent consultant (Lima, Perú). E-mail: a2017200411@uwiener.edu.pe
Iron (Fe) deficiency, in comparison with magnesium (Mg) and manganese (Mn) deficiencies, is an important factor that causes leaf chlorosis when growing arugula hydroponically. In this study, an experimental design was applied, using randomized complete blocks with four groups of six plants each: three experimental groups (EG) and one control group (CG). Precautions were taken to ensure that intervening variables such as artificial oxygenation and time did not affect the outcome of the experiment. The results obtained show that Fe, Mg, and Mn deficiency in each treatment influences the appearance of leaf chlorosis. Likewise, it is evident that the shortage of oxygenation and the excess of macronutrients influence the chlorosis and necrosis of the arugulae, respectively.
Keywords: arugula; chlorosis; Fe; Mg; and Mn deficiency; hydroponic garden; treatment.
INTRODUCTION
This research aims to determine the impact of iron (Fe), magnesium (Mg) and manganese (Mn) on the color of arugula leaves grown in a hydroponic system with the purpose of avoiding the presence of chlorosis and maintaining its organoleptic characteristics such as greenness, size and adequate weight. In these critical times due to population growth (which needs to satisfy a food demand with high quality crops that are beneficial to health), arugula is presented as a good alternative.
In view of this, Sturm and Wagner (2017) argue that high consumption of vegetables belonging to the Brassicaceae family (such as arugula) is related to a lower incidence of chronic diseases (including different types of cancer) due to the glucosinolates (GLS) -sulfur-containing secondary plant compounds that are almost exclusively present in Brassicaceae- and, in particular, to their bioactive degradation products, including isothiocyanates (ITCs), as they are beneficial to health and possess anticancer and anti-inflammatory properties. Marchioni et al. (2021) argue that adult arugulae are mainly composed of isothiocyanates, especially 4-(methylthio)butyl isothiocyanate and nitriles.
However, the industrialization of food forces the acceleration of harvests. Thus, the disproportionate use of insecticides and chemical compounds to combat pests is observed, which affects the soil and food and, consequently, causes damage to people's health.
Hydroponic systems are proposed as an alternative solution to this problem. According to Beltrano and Giménez (2015), they integrate techniques that allow plants to have nutrients, to be far from the soil and to maintain humidity and temperature conditions that allow good growth.
Jordan et al. (2018) studied lettuces grown in aquaponic and hydroponic systems, which obtained higher yields when using substrates composed of coconut husk fiber.
Gruda (2019) adds that switching from a soil to a soilless growing system will make water use more efficient, especially in closed-loop systems with a recirculating water/nutrient solution that recovers drainage water for reuse. On the other hand, Cordeiro et al. (2019) highlight that, in hydroponics, to prepare the nutrient solution and ensure its success in arugula crops, high quality water must be used. The proper use of water and the absence of pesticides in crops reduces harvesting time. To this, Sánchez-Del Castillo et al. (2021) add that properly managed hydroponic crops are ten times more productive than open field crops.
Several institutions in the world dedicated to crop research use hydroponics. The Universidad Nacional Agraria La Molina (UNALM) uses this technique in its hydroponic garden with various agricultural products. It is in this context that arugula or rocket (Eruca sativa), beneficial to the health of our society, was studied. It is necessary to control the chlorosis of this plant to obtain adequate greenery, size and weight at harvest (see Figure 1).
Chlorosis in arugula has several causes. The lack of iron, magnesium and manganese may cause arugula to have yellow, poor condition leaves (see Figure 2) with substandard weights and sizes. This causes losses to farmers growing this plant.
Rodríguez and Flórez (2004) maintain that the macronutrients and micronutrients absorbed by plants are of vital importance and there is a total of 17; these include oxygen, hydrogen and carbon (which come from water, carbon dioxide and air), as well as mineral nutrients. In addition, concentrations above 0.1% of macronutrients such as nitrogen, phosphorus, potassium, calcium, magnesium and sulfur were found in plant tissues in dry weight. The authors mentioned that micronutrients are needed in concentrations below 100 μg/g dry weight.
Silva et al. (2017) conducted a study on nutrient deficiency in which they emphasized the description of macronutrient deficiency symptoms in peppers. The authors determined that the absence of macronutrients such as potassium and magnesium caused chlorosis and leaf necrosis.
In experiments on iron deficiency chlorosis (IDC) for soybean in alkaline soils, Santos et al. (2021) concluded that the application of synthetic iron chelates is the most widely used in IDC amendment. To measure the effect of the iron chelate application for chlorosis treatment, SPAD values were measured at each consecutive phenological stage, from V1 to V5.
Several authors such as Aslani and Souri (2018), Ahmadi and Souri (2018), and Riaz and Guerinot (2021) have studied the importance of iron in plants. They concluded that iron is necessary for physiological processes such as photosynthesis, chlorophyll synthesis, nitrogen fixation, respiration, enzyme activation and electron transfer. That is, iron deficiency can disrupt these processes at different rates and levels.
In their research on arugula, Flores et al. (2014) determined that the increase in population influenced the demand for quality natural foods with high nutritional values.
The scope of this research is exploratory since there are few studies on the deficiencies in arugula and their influence on the quality of this plant cultivated in a hydroponic system. The purpose is to solve problems related to the chlorosis in the leaves of arugula cultivated in hydroponics. The investigation also aims to contribute to the academic debate to contribute to research on anticancer products. It has a social scope since a healthy diet that includes vegetables such as arugula (which contain carotenoids and have antioxidant nutritional properties) is a beneficial alternative for human health. Finally, it has an economic scope, since its low cost and the need for little space make arugula an alternative crop for low-income growers, but it is also possible to initiate investment projects in the agroindustrial business.
For this research, four objectives were established: 1) to determine if the means of dS m of electrical conductivity are different in the four treatments, 2) to determine if the means of leaf size are different in the four treatments, 3) to determine if the means of plant fresh weight (in grams) are different in the four treatments and 4) to determine if the means of plant dry weight (in grams) are different in the four treatments.
Hypotheses
The following hypothesis were proposed:
The dS m means of electrical conductivity are different in the four treatments.
The means of leaf size are different in the four treatments.
The means of plant fresh weight (in grams) are different in the four treatments.
The means of plant dry weight (in grams). are different in the four treatments.
METHODOLOGY
Temporal Scope
For this research, arugula plants were grown in February 2019. The experiment was conducted in March of that year and lasted 30 days.
Spatial Scope
This experiment was conducted in a hydroponic orchard of a national university in the district La Molina, Lima, which is geographically located at 12°04’55” south latitude and 76°56’53” west longitude, and is at 243.7 masl, within the so-called subtropical desiccated desert (dd-S). The average annual temperature in this zone is 20 ºC, with an average relative humidity of 84% and annual rainfall of 11.9 mm. The garden has adequate conditions in regards to shade, relative humidity, and wind strength. The research line of this work is “hydroponic crops”.
Type and Design of Research
The research corresponds to the applied type and its level is relational, since it links the variables in a cause-effect manner. The design is experimental since the independent variable is manipulated in order to observe its influence on the dependent variable. It has a quantitative approach since the results are quantities. Two of its agronomic variables were transversal and two longitudinal.
Population and Sample
For this experiment, the common arugula plant was used. The population consisted of 150 arugula seedlings from the UNALM hydroponic garden.
A sample of 24 arugulae was selected, considering six replicates for four treatments, although, in experimental research, three to four replicates are usually used.
Material instruments
In the nursery bed phase, the following were used: a fruit box 60 cm long, 40 cm wide and 15 cm high; 12 m3 of coarse sand; 10 m of black plastic; a 5 cm long per 0.5 cm diameter microfilm, and a 1 cm2 mesh. In the next phase, the following were used: a pH meter to measure the degree of acidity in a solution; a conductivity meter to measure EC; a 1 L measuring cylinder; a fruit box 60 cm long, 40 cm wide and 15 cm high; a 1” thick sheet of styrofoam; 30 plastic cups of 1 oz; 30 sponges of 1 cm2, and a 1 L measuring jug.
In the second NFT transplant, 20 plastic tubes of 15 m long and 3” in diameter, a 1” distribution piping system, a 1” stopcock, an iron sawhorse and six 5 cm long per 0.5 cm diameter microfilm hoses were used.
In the experimental stage, a 1 L test tube, a hundred A4 sheets, a 30 cm ruler, two pens, 24 plastic pots with a capacity of 2 L each, 24 circular styrofoam lids of 5 cm diameter, 24 plastic keys, 24 water conductors for fish tank motors of 10 cm length per 0.5 cm diameter, 24 Kraft paper bags and 1.6 L of water for each pot were used. A 100-sheet notebook was also used as a diary and field notes.
Equipment
In the NFT stage, a polyethylene tank with a capacity of 1000 L for an area of 100 m2 and an 80 L per minute 0.5 HP electric centrifugal pump was used. In the experiment stage, three fish tank motors, a digital scale, an electronic scale, a drying oven, a calculator, and a HP laptop computer were used.
Nutrient Solution
In the experimental stage, La Molina hydroponic nutrient solution was used, as proposed by Caso et al. (2010), whose components are concentrated solution A, composed of N, P, K and Ca, and concentrated solution B, composed of Mg, S, Fe, Mn, Cu, Zn, B, Mo and Cl. The nursery bed stage had a duration of 15 days and the dose used was 5 ml of solution A per 1 L of water and 2 ml of solution B per 1 L of water. Following the nursery bed stage, a dosage of 3 ml of solution A per 1 L of water and 2 ml of solution B per 1 L of water was used for a duration of 6 days. The final transplant lasted 9 days and the doses used were 5 ml of solution A per 1 L of water and 2 ml of solution B per 1 L of water.
In the second stage of the experiment, a division of four groups was made with six pots of 1.6 L each. The time duration was 30 days, and Fe, Mg and Mn control deficiencies were treated.
In the CG, 3 ml of solution A per 1 L of water was used, thus 4.8 ml per pot and 2 ml of solution B per 1 L of water, corresponding to 3.2 ml per pot.
In the iron-deficient group, all nutrients except iron were used as follows: 3 ml of solution A per 1 L of water, which is a total of 4.8 ml per pot; 1 ml of magnesium sulfate; 1 ml of manganese sulfate; and 1 ml of micronutrients.
In the magnesium-deficient group, all nutrients except magnesium were used as follows: 3 ml of solution A per 1 L of water which is a total of 4.8 ml per pot; 1 ml of iron chelate; 1 ml of manganese sulfate; and 1 ml of micronutrients.
Finally, in the manganese-deficient group, all nutrients except for manganese were used as follows: 3 ml of solution A per 1 L of water which is a total of 4.8 ml per pot; 1 ml of magnesium sulfate; 1 ml of iron chelate; and 1 ml of micronutrients.
Procedure
Twenty-four plants were randomly selected and divided into four groups, with six plants per group (three experimental and one control), in a 1.6 m2 plot area, with one row and one plant per experimental unit. The total area of the experiment was 2.5 m2.
Conditioning
The particulate substrate was moistened with the La Molina hydroponic nutrient solution, as was done by Caso et al. (2010), with 5 ml of solution A and 2 ml of solution B per 1 L of water. Subsequently, the nursery bed stage was initiated where the substrates were placed, with a volume of 12 m3, in a fruit box 60 cm long per 40 cm wide. Then, 8 furrows were drawn with distances of 5 cm and a depth of 1 cm. On day 1, seeds were planted in each row, then covered with the substrates, and then irrigation using only water was started for 5 days. From day 6 to day 10, irrigation was carried out with a 50% concentration of solution and, finally, from day 11 to 15, irrigation was carried out with a 100% concentration of nutrient solution.
The stage following the nursery bed stage lasted 6 days and began with the transplanting of selected plants, whose roots were carefully washed, with the necks of the seedlings covered with a piece of sponge, separately, to be fastened in the hole of the styrofoam, so that the roots were submerged with the nutrient solution for 15 days, as done by Caso et al. (2010). However, this length of time caused chemical burn of the seedlings due to lack of proper oxygenation, as they suffered water stress. It was decided to reduce the process to 5 days since the roots had developed and were ready to be transferred to the NFT system where oxygenation is mechanical.
Caso et al. (2010) also proposed doses of 5 ml of solution A and 2 ml of solution B, but 2 ml of solution A was reduced because the chemical elements that compose it had a high concentration of salts that caused the seedlings to burn due to water stress.
It was found that arugula plants are sensitive when oxygen is scarce and, to solve this restriction, fish tank motors were placed in each pot. The experimental stage lasted 30 days and 24 plants were selected and randomly distributed. Then, all the nutrient solutions of the control group and the three nutrient solutions with iron, magnesium and manganese deficiencies were prepared. Finally, the 24 pots divided into 4 groups were conditioned and 1.6 L of solution was added to each pot with their respective oxygen conduits attached to the fish tank motor.
Sixty days after planting began, the 24 plants were harvested. They were measured and weighed and then dried in an industrial oven. The day after the harvest, 24 samples of roots and 24 samples of leaves were placed in Kraft paper bags and placed in the industrial oven at a temperature of 95 °C. Finally, the 24 pairs were weighed.
RESULTS
The samples were evaluated considering electrical conductivity (EEC), leaf size, fresh weight, and dry weight of the plant. Table 1 shows the results obtained.
Table 1 Average Values for Each Experimental Variable and its P-Value.
Experimental variables | Control Treatment | Iron Deficiency Treatment | Magnesium Deficiency Treatment | Manganesium Deficiency Treatment | p-value |
---|---|---|---|---|---|
EC in dS m | 1.6 | 1.5 | 1.13 | 1.22 | 0.000 |
Leaf size in cm | 25.5 | 15 | 13.33 | 12.67 | 0.000 |
Fresh weight in g | 93.67 | 31.67 | 23.33 | 35.17 | 0.000 |
Dry weight in g | 8.44 | 3.38 | 2.87 | 2.97 | 0.000 |
Source: Prepared by the author.
Table 2 shows the values resulting from Tukey's test for each experimental variable for paired comparisons of means.
Table 2 Tukey's Test for Each Experimental Variable.
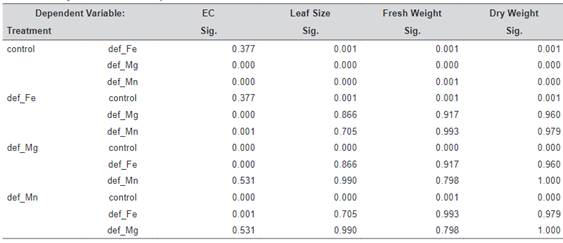
The mean difference is significant at the 0.05 level.
Source: Prepared by the author.
Electrical Conductivity (EC)
The results obtained for this variable are shown in Table 1. The control group, which included Fe, Mg and Mn, had an average of 1.6 dS m (density of salts per meter).
The treatment corresponding to iron deficiency has the highest mean (1.5 dS m) with respect to the other deficiencies (magnesium and manganese). This means that iron deficiency causes a high EC or salt concentration compared to magnesium deficiency (whose average is 1.13 dS m) and manganese deficiency (whose average is 1.22 dS m).
The null hypothesis (H0) states that, the means of dS m of EC are the same in the four treatments. The statistical test used was Analysis of Variance (Anova), the significance level was 0.05 and the p-value was 0.000, as shown in Table 1. Then, if p-value is less than 0.05, the H0 is rejected, at least one of the means of the treatments is different.
Table 2 shows Tukey’s test for paired comparisons of means. The results indicate that the means of the control and iron deficiency treatment samples are equal; the same is true for the means of the magnesium deficient and manganese deficient samples, which are also the same.
Control and iron deficiency treatments are more effective for CEE; control treatment is slightly more effective than iron deficiency treatment.
Leaf Size
Table 1 shows that, for this experimental variable, the mean of the leaf size for the control treatment was 25.5 cm, the largest. On the other hand, the size of the leaves corresponding to the iron deficiency treatment had an expected value of 15 cm; however, the manganese deficiency treatment had the smallest size, reaching an average of 12.67 cm.
The null hypothesis (H0) states that the means of leaf size are the same in the four treatments. The statistical test used was Analysis of Variance (Anova), the significance level was 0.05 and the p-value was 0.000, as shown in Table 1. Then, if p-value is less than 0.05, H0 is rejected, at least one of the means of the treatments is different.
Table 2 shows the Tukey’s test for paired comparisons of leaf size means. It is observed that the control treatment mean is not equal to the means of Fe, Mg, and Mn deficiencies; however, it is observed that the means of iron, magnesium and manganese deficiencies are equal with each other. Therefore, the control treatment is more effective than the other treatments.
Plant Fresh Weight (PFW)
Table 1 shows the weights obtained during harvest. The control treatment, which contains Fe, Mg, and Mn, has the highest average, 93.67 g, which means that the weight will be higher when there is no deficiency of iron, magnesium and manganese. The second best result was obtained in treatment 3, which had manganese deficiency with 35.17 g, which means that this element is not influential in the final fresh weight of arugula. It is also observed in Table 1 that the treatment with magnesium deficiency resulted in an average weight of 23.33 g, that is, the lowest average fresh weight, indicating that this element is the most important factor.
The null hypothesis (H0) states that the means of plant fresh weight (in g) are equal in the four treatments. The statistical test used was Analysis of Variance (Anova), the significance level was 0.05 and the p-value was 0.000, as shown in Table 1. Then, if p-value is less than 0.05, H0 is rejected, at least one of the means of the treatments is different.
Table 2 shows the Tukey’s test for paired comparisons of plant fresh weight means. It is observed that the control treatment mean is not equal to the means of Fe, Mg, and Mn deficiencies; however, it is observed that the means of iron, magnesium and manganese deficiencies are equal with each other. Therefore, the control treatment is more effective than the other treatments.
Plant Dry Weight (PDW)
After drying the plants, they were weighed. The average of the treatments expressed in g is shown in Table 1. It is observed that the control treatment, containing Fe, Mg, and Mn, presents the highest average, 8.44 g, that is to say, it is the most effective. It is followed by the treatment with iron deficiency with an average value of 3.38 g. On the other hand, the treatment with magnesium deficiency has the lowest average with a weight of 2.87 g.
The null hypothesis (H0) states that the means of plant dry weight (in g) are equal in the four treatments. The statistical test used was Analysis of Variance (Anova), the significance level was 0.05 and the p-value was 0.000, as shown in Table 1. Then, if p-value is less than 0.05, H0 is rejected, at least one of the means of the treatments is different.
Table 2 shows the Tukey’s test for paired comparisons of plant dry weight means. It is observed that the control treatment mean is not equal to the means of Fe, Mg, and Mn deficiencies; however, it is observed that the means of iron, magnesium and manganese deficiencies are equal with each other. Therefore, the control treatment is more effective than the other treatments.
DISCUSSION
Electrical Conductivity (EC)
The results showed that the EC in the control treatment reached an average of 1.6 dS m, which agrees with the findings of the following studies:
Da Silva et al. (2011) conducted research on arugula crops that were subjected to different salinity levels in NFT hydroponic culture, where subway saline water and its desalination residues were used. Six salinity levels were used (water EC of 0.2; 1.2; 2.2; 3.2; 4.2 and 5.2 dS m-1) with randomized treatments in a 6 x 2 factorial, with four replications. They also point out that, from transplanting, arugula plants were evaluated for visual detection of symptoms, with no toxicity or nutritional deficiencies resulting from imbalances caused by salts up to 12 days after transplanting. No symptoms were recorded in plants subjected to salinity of subway origin (saline water).
However, crops that were subjected to higher salinity levels by NaCl showed symptoms of chlorosis, regardless of the salt source, as they presented chlorotic leaves and, eventually, marginal leaf burn. The symptoms produced by the saline water were less intense than the symptoms produced by the NaCl source. But, for both sources, no symptoms were detected in plants subjected to salinity of 1.2 dS m-1, whose appearance was similar to that of the control treatment plants (ECa = 0.2 dS m-1). Chlorosis was the most evident symptom in the plants, with a greater predominance on their edges, especially at the higher salinity levels (ECa = 4.2 and 5.2 dS m-1).
On the other hand, Carassay et al. (2018) supported the possibility of achieving adequate yield and high quality in arugula by controlling salinity problems. An open field investigation was conducted in the Plant Physiology laboratory with three arugula varieties (Sais, Bonanza and Florensa) that were imported. Sodium chloride and bicarbonate or a mixture of these two elements were used. The various types of arugula showed high quality in the salinized lot (14.5 dS.m-1). High yields were within the range of 12,000 to 20,000 kg.ha-1. The first 20 cm of the plot was classified as saline because it had an EC of 14.5 dS.m-1 and a slightly alkaline pH of 7.5.
Dos Santos et al. (2020), based on studies on arugula yield, argue that the use of shading together with irrigation water salinity up to 1.4 dS m-1 does not affect the leaf area of arugula plants, so it is possible to grow without considerable losses when referring to the electrical conductivity of irrigation water.
In this research it was found that the control treatment containing the iron, magnesium and manganese components obtained the highest average of 1.6 dS m, a value within the range found by the aforementioned researchers. There is a risk of chemical burn of the leaves when the value of 2 dS m is exceeded.
Leaf Size
The results showed that the size of the arugula leaves in the control treatment reached an average of 25.5 cm in height, as observed in the following research findings:
Del Pino (2012) indicates that these plants can have a height equal to or greater than 25 cm. Da Silva et al. (2019) find that arugula production in Brazil, using seeds produced in the conventional system, obtain higher values in terms of plant height in both summer and autumn in the cultivars Ágatha (with 30.3 cm and 30.4 cm), Astro (29.4 cm and 31.3 cm) and Giovana (30 cm and 31.1 cm).
The results also showed that the control treatment containing iron, magnesium and manganese components obtained the best average of 25.5 cm, which is within the parameters of the previous findings. The results also showed that, with manganese deficiencies, leaf size on average reached a growth of 12.67 cm, being of the lowest growth within the treatments.
Plant Fresh Weight (PFW)
The results also showed that the control treatment containing the Fe, Mg, and Mn components obtained the best average regarding FPW, which was 93.67 g. The findings of Jesus et al. (2015) in hydroponic arugula cultures under salt stress found that increased salinity reduced dry weight, with the mean values for long leaf cultures being 39.73 g and cultivated leaf 36.28 g; the former is observed to be superior, which coincides with higher dry biomass production in leaves and shows the effectiveness of the antioxidant defense system in maintaining genotype growth in the face of increased salinity in the culture solution.
Schmidt et al. (2017) evaluated the impact of various cultivation profile colors in NLT with respect to microclimate, growth and yield of four arugula cultivars, in two seasons, and obtained the highest average PFW with the Cultivar technique, which was 2394.01 g/m2.
In their study on NO3 -/NH4 + ratios in lettuce crops, Lara et al. (2019) obtained on average a PFW of 268.87 g in a ratio of 80/20; a PFW of 255.38 g in a ratio of 65/35; and a FPW of 240.44 g in a ratio of 50/50. The mixture of NO3 - and NH4 + was adequate, the summer and autumn seasons did not affect lettuce growth and there were no significant differences between the two seasons.
For the Gionava cultivar PFW, Cordeiro et al. (2019) obtained the best performance of arugula crops with an organic system both in autumn, with an average value of 34.6 g, and in summer, with an average value of 44 g. Guardabaxo et al. (2020) found better developments in the cultivation of arugula with the use of salt concentrations between 67% and 100%. Thus, 617.9 g and 655.8 g were obtained for PFW, respectively, and did not differ among the different treatments.
Plant Dry Weight (PDW)
The results also showed that the control treatment, containing Fe, Mg, and Mn, obtained the best average in terms of PDW, which was 8.44 g. In the findings of Jesus et al. (2015) on hydroponic arugula cultivars under salt stress, it was observed that increased salinity reduced fresh weight, where the mean values for Longleaf were 14.33 g, and Cultivata, 11.12 g. Thus, the former had a higher result, which coincided with a higher production of fresh plant biomass in leaves, which demonstrates the effectiveness of the antioxidant defense system that maintains the growth of the genotype in the face of increased salinity in the culture solution.
In their study on NO3 -/NH4 + ratios in lettuce crops, Lara et al. (2019) obtained on average a PDW of 20.34 g in a ratio of 80/20; a PDW of 18.42 g in a ratio of 65/35; and a PDW of 17.40 g in a ratio of 50/50. The mixture of NO3 - and NH4 + was adequate, the summer and autumn seasons did not affect lettuce growth and there were no significant differences between the two seasons. Da Silva et al. (2019) studied arugula cultivars produced in a protected organic system in both summer and fall. Average dry weight yields were found to be higher in summer with the cultivars Agatha (2.5 g) and Giovana (2.53 g) while, in autumn, Giovana obtained the highest average with 2.22 g and Cultivated, 2.19 g.
Riaño et al. (2019) made nutrient modifications to a hydroponic solution used for baby spinach, in this case the solution “La Molina”, and obtained in one of their replicates the highest average of 1.69 g. Guardabaxo et al. (2020) found that concentrations of salts above 67 %, whose average weight was 32.32 g, did not produce significant increases in PSW.
CONCLUSIONS
The cultivation of arugula plants through the hydroponics system is a pesticide-free, water-based cultivation without the use of soil, which generates higher productivity. Arugula, due to its properties, is a beneficial plant for good health, as this vegetable is related to a lower incidence of chronic diseases (including different types of cancer) thanks to its glucosinolates, which contain sulfur, and, in particular, to its bioactive degradation products (including isothiocyanates).
The results of this research are intended to encourage investment projects in the agroindustrial sector, specifically in the cultivation and processing phases, whether as a fresh, dried or encapsulated product. Consequently, it is necessary to promote the benefits of arugula to society and encourage its consumption.
Another importance of this research is to provide the necessary care for the cultivation of this plant in the hydroponic system and thus determine which elements influence the maintenance of the beneficial properties of arugula for humans. To avoid chlorosis, the presence of iron is determinant, followed by magnesium and manganese to maintain the organoleptic qualities of arugula.
During the experiment there were intervening factors: 1) It was necessary to reduce the dose of macronutrients from 5 ml to 3 ml/L of water. In the case of the micronutrients Fe, Mg, and Mn, the dose of 2 ml/L of water was maintained; 2) The EC was controlled within the range of 1-2 dS m in order to avoid chlorosis due to chemical burn; 3) The duration of the post nursery bed stage was reduced from 15 to 6 days to avoid chlorosis due to deficient oxygenation in the roots; 4) In the experimental process, it was observed that the roots of the 24 experimental units required proper oxygenation, so artificial oxygenation was necessary through the use of fish tank motors.
Finally, in the experiment it was observed that the roots of arugula were U-shaped.
ACKNOWLEDGMENT
To the workers of the hydroponic garden of the UNALM, who helped with this research.
REFERENCES
Ahmadi,M., y Souri, M. K. (2018). Growth and mineral content of coriander (Coriandrum sativum L.) plants under mild salinity with different salts. Acta Physiologiae Plantarum, 40(11),1-8. https://doi.org/10.1007/s11738-018-2773-x [ Links ]
Aslani,M., y Souri, M. K. (2018). Growth and Quality of Green Bean (Phaseolus vulgaris L.) under Foliar Application of Organic-Chelate Fertilizers.Open Agriculture, 3(1),146-154. https://doi.org/10.1515/opag-2018-0015 [ Links ]
Beltrano,J., y Giménez,D. (2015). Cultivo en hidroponía. Buenos Aires, Argentina: Editorial de la Universidad Nacional de La Plata. https://libros.unlp.edu.ar/index.php/unlp/catalog/book/414 [ Links ]
Carassay, L., Ponce, J., Siliquini, O., Bartel, A., Kin, A., Minig, M., Moyano, J., Ahumada, G., Baudino, E., y Taleisnik, E. (2018). Efecto de la salinidad en el cultivo de (Eruca sativa) “rúcula” cultivada en invernadero en la provincia de La Pampa. Semiárida, 25(2), 53-54. https://redib.org/Record/oai_articulo2793917-efecto-de-la-salinidad-en-el-cultivo-de-eruca-sativa-“rúcula”-cultivada-en-invernadero-en-la-provincia-de-la-pampa [ Links ]
Caso, C., Chang, M., y Rodríguez-Delfín, A. (2010). Efecto del sustrato sobre la producción de fresa en sistema de columna. Red Hidroponía, Boletín Núm. 46, 7-12. https://docplayer.es/20945068-Red-hidroponia-boletin-no-46-2010-lima-peru.html [ Links ]
Cordeiro, C. J. X., Neto, J. de S. L., de Oliveira, M. K. T., Alves, F. A. T., Miranda, F. A. da C., y de Oliveira, F. de A. (2019). Cultivo de rúcula em fibra de coco utilizando solução nutritiva salinizada enriquecida com nitrato de potássio. Revista Brasileira de Agricultura Irrigada, 13(1), 3212-3225. https://doi.org/10.7127/rbai.v13n100960 [ Links ]
Da Silva, A., da Silva, D., Soares, T., Silva, Ê., dos Santos, A., y Rolim, M. (2011). Produção de rúcula em sistema hidropônico NFT utilizando água salina do Semiárido - PE e rejeito de dessalinizador. Agrária, 6(1), 147-155. https://doi.org/10.5039/agraria.v6i1a929 [ Links ]
Da Silva, P. A., Kinjo, S., de Melo, M. P. B. X., y Sala, F. C. (2019). Evaluation of arugula cultivars and seed production in the organic system. Journal of Seed Science, 41(4), 423-430. https://doi.org/10.1590/2317-1545v41n4218457 [ Links ]
Del Pino, M. (2012). El cultivo de rúcula. Contacto Rural(1), 10-11. http://sedici.unlp.edu.ar/handle/10915/62543 [ Links ]
Flores, M., Martínez, M., Rodríguez, J., Colinas, M., y Nieto, D. (2014). Jugo de brócoli en la inhibición de alternaria alternata en arúgula mínimamente procesada: calidad postcosecha. Revista Chapingo. Serie horticultura, 20(3), 307-322. https://doi.org/10.5154/r.rchsh.2013.09.028 [ Links ]
Gruda, N. (2019). Increasing Sustainability of Growing Media Constituents and Stand-Alone Substrates in Soilless Culture Systems. Agronomy, 9(6), 298. https://doi.org/10.3390/agronomy9060298 [ Links ]
Guardabaxo, C., Assis, K., Figueiredo, F., y Silva, L. (2020). Cultivo da rúcula em sistema hidropônico sob diferentes concentrações de sais. Brazilian Journal of Biosystems Engineering, 14(3), 274-282. https://doi.org/10.18011/bioeng2020v14n3p274-282 [ Links ]
Jesus, C., Silva, F., Camara, T., Silva, Ê., y Willadino, L. (2015). Production of rocket under salt stress in hydroponic systems. Horticultura Brasileira, 33(4), 493-497. https://doi.org/10.1590/S0102-053620150000400014 [ Links ]
Jordan, R., Ribeiro, E., Oliveira, F., Geisenhoff, L., y Martins, E. (2018). Yield of lettuce grown in hydroponic and aquaponic systems using different substrates. Revista Brasileira de Engenharia Agrícola e Ambiental, 22(8), 525-529. https://doi.org/10.1590/1807-1929/agriambi.v22n8p525-529 [ Links ]
Lara, A., Rojas, A., Romero, M., Ramírez, H., Cruz, E., Alcalá, J., y Loredo, C. (2019). Crecimiento y acumulación de NO3- en lechuga hidropónica con relaciones nitrato/amonio en dos estaciones de cultivo. Revista Fitotecnia Mexicana, 42(1), 21-29. http://www.scielo.org.mx/scielo.php?pid=S0187-73802019000100021&script=sci_abstract [ Links ]
Marchioni, I., Martinelli, M., Ascrizzi, R., Gabbrielli, C., Flamini, G., Pistelli, L., y Pistelli, L. (2021). Small Functional Foods: Comparative Phytochemical and Nutritional Analyses of Five Microgreens of the Brassicaceae Family. Foods, 10(2), 427. https://doi.org/10.3390/foods10020427 [ Links ]
Riaño, E., Caicedo, L., Torres, A., y Hurtado, H. (2019). Cambios en los niveles de nutrientes en solución hidropónica de espinaca baby (Spinacia oleracea L.), para su futura aplicación en acuapónia. Orinoquia, 23(1), 73-84. https://doi.org/10.22579/20112629.544 [ Links ]
Riaz, N., y Guerinot, M. (2021). All together now: regulation of the iron deficiency response. Journal of Experimental Botany, 72(6), 2045-2055. https://doi.org/10.1093/jxb/erab003 [ Links ]
Rodríguez, M., y Flórez, V. (2004). Elementos esenciales y beneficiosos. (Monografía). Universidad de Almería, Almería. http://repositorio.ual.es/handle/10835/3133#.YhxO-lcjERc.mendeley [ Links ]
Sánchez-del Castillo, F., Portillo-Márquez, L., Moreno-Pérez, E., Magdaleno-Villar, J., y Vázquez-Rodríguez, J. C. (2021). Effects of container volume and seedling density on late transplanting and number of flowers in tomato. Revista Chapingo, Serie Horticultura, 27(2), 71-84. http://dx.doi.org/10.5154/r.rchsh.2020.06.015 [ Links ]
Santos, C. S., Rodrigues, E., Ferreira, S., Moniz, T., Leite, A., Carvalho, S. MP., Vasconcelos, M. W., y Rangel, M. (2021). Foliar application of 3-hydroxy-4-pyridinone Fe-chelate [Fe(mpp)3] induces responses at the root level amending iron deficiency chlorosis in soybean. Physiologia Plantarum, 173, 235-245. https://doi.org/10.1111/ppl.13367 [ Links ]
Schmidt, D., Gabriel, V., Caron, B., Souza, V., Boscaini, R., Pinheiro, R., y Cocco, C. (2017). Hydroponic rocket salad growth and production according to different color profiles. Horticultura Brasileira, 35(1). https://doi.org/10.1590/S0102-053620170117 [ Links ]
Silva, A., Arderson, F., Nowaki, R., Cecilio, A., y Mendoza-Cortez, J. (2017). Síntomas de deficiencia de macronutrientes en pimiento (Capsicum annuum L.). Agrociencia Uruguay, 21(2), 31-43. http://www.scielo.edu.uy/scielo.php?script=sci_arttext&pid=S2301-15482017000200031&lng=en&tlng=en [ Links ]
Dos Santos, R., dos Santos, M., da Silva, F., Santos, J., dos Santos, S., Reis, L., y Tavares, C. (2020). Desempenho da rúcula sob condições de sombreamento e níveis de salinidade da água de irrigação. Colloquium Agrariae, 16(4), 38-45. https://doi.org/10.5747/ca.2020.v16.n4.a381 [ Links ]
Sturm, C., y Wagner, A. (2017). Brassica-Derived Plant Bioactives as Modulators of Chemopreventive and Inflammatory Signaling Pathways. International Journal of Molecular Sciences, 18(9), 1890. https://doi.org/10.3390/ijms18091890 [ Links ]
Received: September 09, 2022; Accepted: October 17, 2022