Introduction
The use of PGPB is an environment friendly, low-cost alternative that is being implemented in a variety of crops. Nevertheless, the implementation of this technology is very limited, despite being known for decades. Consequently, the use of microorganisms as biofertilizers is not a widespread practice. This is due to the particular use conditions of each microbial group that can be considered as PGPB (Vejan et al., 2016; Tabassum et al., 2017).
The use of living organisms that interact with plants favors the development through direct and indirect mechanisms, which have been described by different researchers (Brader et al., 2014; de Souza et al., 2015). These mechanisms are based on the ability to produce phytohormones and siderophores, and to solubilize phosphates or other important nutrients for the plant. Indirect mechanisms are related to the production of antimicrobial compounds that prevent the attack of pathogens, induce systemic resistance on the plant, and improve soil conditions (Bishnoi, 2015). Therefore, applying biofertilizers would reduce the use of chemical products that have generated a severe environmental impact (Bouraoui and Grizzetti, 2013).
Gluconacetobacter diazotrophicus is a very efficient nitrogen-fixing bacterium with which many interaction studies have been carried out with several non-legume plants (Oliveira et al., 2009; Luna et al., 2012; Chawla et al., 2014). The flexibility in the interactions between this bacterium and different vegetables makes it a very attractive alternative as a biofertilizer. G. diazotrophicus has exhibited the ability to colonize different plants and can be inserted in different tissues. It was isolated initially in sugarcane crops, but it has also been isolated in tomato (Luna et al., 2012), rice (Jha et al., 2009), corn (Tian et al., 2009) and a variety of tropical and subtropical plants (Madhaiyan et al., 2004). In the same way, this bacterium has been detected in plant organs such as stems, leaves, fruits, and seeds (Botta et al., 2013; Santoyo et al., 2016).
The ability to infect the internal tissues of the root, as well as other organs, allows the bacteria to generate a direct benefit to the host, and to avoid competition with soil and rhizosphere microorganisms. It has been described the bacterial mechanisms to be able to colonize plant tissues, and their mobilization through the vascular tissues of the plant to reach other organs (Gaiero et al., 2013). This bacterium has shown favorable results for plant development under different conditions, either inoculated individually or in consortium with other bacteria (Hernández-Escareño et al., 2015). There are also other species within the family and the genus that possibly have similar promoter properties to G. diazotrophicus (Muthukumarasamy et al., 2005; Mehnaz et al., 2006). The objective of this work was to isolate native G. diazotrophicus from sugarcane located in different regions of northern Peru, as well as test their potential as a biofertilizer.
Materials and methods
Isolation and phenotypic characterization
Samples were collected from the northern coast of Peru, in Ancash, La Libertad, Lambayeque and Piura Regions. Each plant sample was subdivided into leaf, stem, and root. 10 g of each subsample was taken and disinfected with 70% ethanol, then with a 2% sodium hypochlorite solution for 5 min, and consecutive washes with sterile distilled water. Then the samples were macerated and diluted up to 10-3 in a sterile solution of NaCl (0.8%). 100 μl of each dilution was inoculated in the semisolid LGI-P medium. Incubation was carried out for 5 to 7 days at 30 °C. Then, it was plated on solid LGI-P medium and incubated at 30 °C for 5 to 7 days (Baldani et al., 2014).
The phenotypic characterization, catalase, indole, nitrate reduction, and urease were tested. The selected cultures were cultivated in 10% Sucrose Potato Agar (SPA) to observe the dark brown colored colonies and the development capability in LGI-P with 30% sucrose was evaluated (Madhaiyan et al., 2004; Mehnaz et al., 2006).
Amplification of the 16S rRNA gene by PCR and sequencing
The selected isolates were cultured in DYGS medium at 30 °C for 24 hours. Thermal lysis was performed to obtain bacterial DNA. 10 μL of culture was suspended in 100 μL of ultrapure water, and after lysis, the DNA was kept at -20 °C until its use (Rivas et al., 2001). For the amplification of the 16S rRNA gene, Master Mix 2X (Promega) was used according to the manufacturer's instructions. The primers 27F (5'-AGAGTTTGATCCTGGCTCAG-3') and 1492r (5'-GGTTACCTTGTTACGACTT-3') were used at a final concentration of 0.5 μM and 1 μL of the sample was added. PCR was performed in a MasterCycler Eppendorf thermal cycler with a 3 min PCR program at 95 °C, followed by 30 cycles of 95 °C for 30 s, 54 °C for 30 s and 72 °C for 90 s, with a final extension of 10 min at 72 °C. PCR products were then sent to sequence to Macrogen (Korea), the sequences were ensembled and analyzed using the BLAST tool (http://www.ncbi.nlm.nih.gov/BLAST), to compare the homology of the nucleotide sequences. Related sequences were obtained, and an alignment was made with ClustalW using the software MEGA7. The phylogenetic analysis was done using the Neighbor-Joining method and the Kimura two-parameter substitution model with 1000 Bootstrap replications (Khan et al., 2014).
Multiplex PCR assay for G. diazotrophicus identification
The multiplex PCR technique was developed according to Madhaiyan et al. (2004). Specific primers AC (5'-CTG TTT CCC GCA AGG GAC-3 ') and DI (5'-GCG CCC CAT TGC TGG GTT-3') were used, which amplify a specific region of the 16S rRNA gene in G. diazotrophicus. The second pair of primers used was RB (5'-AGA GTT TGA TYM TGG CTC AG-3') and RM (5'-GGA CTA CCA GGG TAT CTA ATC C-3'), which amplify a region of the 16S rRNA gene in all Bacteria. Reactions of 20 μL were prepared, using a concentration of 0.5 μM for each primer and 1 μL of the sample. The PCR conditions were 3 min at 95 °C, followed by 30 cycles of 95 °C for 30 s, 58 °C for 30 s and 72 °C for 45 s, with a final extension at 72 °C for 5 min. PCR products were visualized on a 1.5% agarose gel stained with ethidium bromide.
Phosphate and zinc solubilization assay
To evaluate the phosphates solubilization ability, the medium developed by the National Botanical Research Institute's Phosphate (NBRIP) was used, using glucose as a carbon source. Incubation was performed at 30 °C for 3 days and then measurements were made of the solubilization zone to establish the solubilization rates of each culture (Singh et al., 2015). For the zinc solubilization test, the NBRIP medium was modified by adding ZnO (0.12%) instead of TCP (Saravanan et al., 2007). The evaluation of the solubilizing ability was carried out in the same way as for phosphates.
Phytohormones production
The production of IAA was evaluated using LGIP medium with tryptophan (0.5 g/L). Incubation was performed for 48 hours at 30 °C. The cultures were centrifuged at 10,000 RPM for 5 min. 250 μL of culture supernatant was mixed with 500 μL of Salkowski reagent, keeping it in darkness for 30 min. The analysis was made in a UviLine 9400 UV/VIS spectrophotometer (Schott) at 530 nm. The uninoculated medium was used as control and a calibration curve was made with IAA (Sigma) (Grobelak et al., 2015).
Salinity tolerance test
Peptone broth was prepared with NaCl concentrations of 0, 0.25, 0.5 and 1%. Incubation was performed for 3 days at 30 °C and the presence of microbial growth was observed by measuring absorbance at 600 nm (Tejera et al., 2003).
Antifungal activity
Bacterial cultures were cultivated on plates with PDA. Four equidistant lines were inoculated at the ends of the plate and incubated for 48 hours at 30 °C. Fusarium sp., Alternaria sp., Roselinia sp., Lasiodiplodia sp., and Sclerotinia sp. 5-day cultures were taken and inoculated in the center of the plates. It was incubated at 25 °C until the development of the fungi in the control plate reached the bacterial inoculation zone. To determine the inhibition percentage, this formula was used: % inhibition = ((RT-RC) / RT) x 100; where RT: Radio Control of the fungus; RC: Radio of the fungus faced with the bacteria (Mohamad et al., 2018).
Plant inoculation
Bacteria were cultivated on 2% sucrose potato medium and then eight bacterial treatments were established: T1: LASFB 1740, T2: LASFB 1573, T3: LASFB 1574, T4: LASFBC 461, T5: LASFBC 1911, T6: LASFB 1740 + LASFB 1643 + LASFB 002, T7: LASFB 1573 + LASFBP 086 + LASFB 009, T8: LASFBC 461 + LASFB 1643 + LASFBP 086. Kosakonia sp. LASFB 1643, Klebsiella sp. LASFBP 086, Enterobacter sp. LASFB 009 and Bacillus sp. LASFB 002, belong to laboratory bacterial collection. The control treatments were established considering one with an uninoculated medium and the second with water. 1 ml of each 0.2 OD600 suspensions or control was added to 30 ml of 1/10 MS solution. In vitro sugarcane seedlings variety Mex73-523 were inoculated in the pre-acclimation stage. Seedlings were immersed in respective treatment for 30 minutes. The seedlings were transferred to trays filled with peat and were evaluated after 60 days.
Results and discussion
Phenotypic and molecular characterization
Five bacteria with the phenotypic character-ristics described for G. diazotrophicus were isolated: LASFB 1573, LASFB 1574, LASFB 1740, LASFBC 461 and LASFBC 1911, observing development of orange colonies in LGIP medium (Figure 1), and brownish-colored colonies in SPA. The results of the biochemical tests were also the same as those reported for the reference strain (Gillis et al., 1989; Madhaiyan et al., 2004), and the presence of a specific amplification product of approximately 445 bp was observed when Multiplex PCR was carried out that confirm de molecular identification.
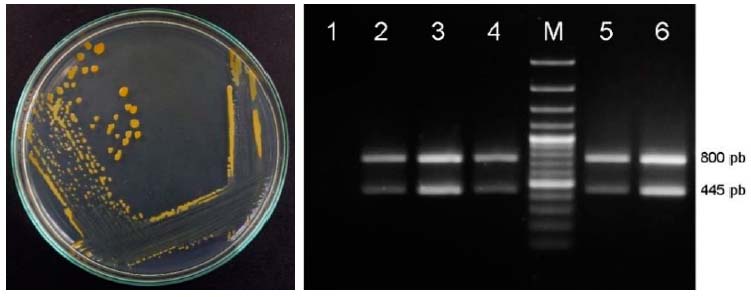
Figure 1 Right. Isolate LASFB 1740 in LGIP medium. Left. Multiplex PCR for G. diazotrophicus identification. Lane 1. PCR control, 2. LASFB 1573, 3. LASFB 1574, 4. LASFB 1740, 5. LASFBC 461, 6. LASFBC 1911, M. Ladder marker.
Table 1 Results of physiological tests of G. diazotrophicus isolated from sugarcane
Bacteria code | Phosphate solubilization index | Zinc Solubilization index | Indole Acetic Acid production (µg/ml) | Saline Stress Tolerance (NaCl) | |||
0% | 0.25% | 0.5% | 1% | ||||
LASFB 1573 | 2.22 | 4.27 | 65.71 | + | + | + | + |
LASFB 1574 | 2.17 | 9.83 | 76.14 | + | + | + | + |
LASFB 1740 | 2.73 | 3.83 | 71.47 | + | + | + | + |
LASFBC 461 | 1.71 | 8.00 | 34.44 | + | + | + | + |
LASFBC 1911 | 2.30 | 5.80 | 21.50 | + | + | + | + |
+: Positive growth; -: Negative growth.
The bacteria were isolated from leaf and stem samples, and no isolation at the root level was attained that demonstrate the skill of this bacterium to move to different plant tissues, which has been observed in other vegetables such as corn (Tian et al., 2009) and other crops considered subtropical (Madhaiyan et al., 2004). However, it was also noted a low number of isolates, which could be related to an absence of this bacteria in the tissues of plants, or perhaps to a low cellular concentration of the same due to the high fertilization rates used on the field. Some authors have described that there is an inverse relationship between fertilization and the presence of nitrogen-fixing bacteria, demonstrating that in the presence of nitrogen fertilizers the population of G. diazotrophicus is considerably reduced (Muthukumarasamy et al., 2006; Rodríguez-Andrade et al., 2015). There is the possibility that the varieties evaluated are not fully compatible with the bacteria. A study in Cuba conducted by Rojas et al. (2012), showed that from 18 varieties of sugarcane, it was only possible to isolate the bacteria in 13 of them (Rojas et al., 2012), and it was not possible to isolate them from sugarcane in a study carried out in Uruguay (Taulé et al., 2012). The analysis of varieties was not considered in this study.
Plant growth promotion activity
Isolated G. diazotrophicus have a moderate ability to solubilize phosphates, with an index of up to 2.73. Their presence allowed the plants access to this important phosphate source and improved their growth (Delaporte-Quintana et al., 2017). The solubilization of these phosphates has been associated with the production of gluconic acids derived from metabolism, which are excreted into the environment, favoring the availability of phosphates for the plant (Intorne et al., 2009).
The solubilization of zinc was higher than phosphate, observing an index of 9.83 for the isolate LASFB 1574. Equally, the solubilization of zinc is considered important, because in some cases this micronutrient is not available to be taken up by the plant. The results show that all the isolates were able to solubilize ZnO in proportions similar or slightly higher than what was reported by Saravanan et al. (2007), who also demonstrated that this bacterium solubilizes other sources of zinc (Saravanan et al., 2007). Intorne et al. (2009) in 2009 demonstrated that phosphate and zinc solubilization processes would be related to the same genes and Saravanan et al. (2007) proposed that this property could influence resistance to M. incognita infections in tomato plants.
Regarding the capability to produce IAA, slightly variable values were observed between 21.5 μg/ml and 71.47 μg/ml. These isolates have a considerable high IAA level production, compared to reported before (Madhaiyan et al., 2004). Saline Stress Tolerance was evaluated, showing that the isolates were able to develop up to a concentration of 1% NaCl (Table 1). The isolates showed tolerance to different NaCl concentrations, which allows us to evaluate the microbial ability to survive in hostile environments, as well as the possibility of helping the plant to have better development in the same conditions, as it has been demonstrated in other studies done with different bacteria (Bhardwaj et al., 2014). This salt stress resistance could be related to the production of ACC deaminase or exopolysaccharides (Khamwan et al., 2018). However, it was observed that the increase in NaCl concentration affects the physiological properties of these bacteria (Tejera et al., 2003).
Antagonistic activity
G. diazotrophicus antagonistic tests have been carried out before on fungi such as Colletotrichum, Fusarium, and Helminthosporium (Muthukumarasamy et al., 2000; Chawla et al., 2014). G. diazotrophicus isolated in this study showed activity against Alternaria sp., Roselinia sp., Lasiodiplodia sp., or Sclerotinia sp.; however, the inhibition was lower to Sclerotinia sp., even nil on LASFB 1573 and LASFB 1740. The isolates showed a percentage of inhibition like the 73.62% reported by Logeshwarn et al. (2011), against Fusarium oxysporum, suggesting that different species of the genus Fusarium may be inhibited by this bacterial action. These results are similar with observed on some genre Bacillus strains that have shown inhibition results for Sclerotinium up to 81.9% (Kamal et al., 2015), 58.9% for Lasiodiplodia (Chukeatirote et al., 2018), and 62.9% for Alternaria (Sharma and Sharma, 2008). Some authors have proposed the synthesis of gluconic acids as antifungal compounds that diffuse in the culture medium (Bertini et al., 2014), and bacteria could also be controlled by the production of gluconacin that has shown a growth inhibition against pathogenic plant-bacteria (Oliveira et al., 2018). In contrast, others have evaluated the production of volatile compounds that would have a similar effect (Meena et al., 2017).
Plant inoculation test
The inoculation experiments carried out with the bacterial isolates show that they are all capable of improving the plant development of sugarcane plants. The inoculation of treatments T5 and the mixture of T7 were the treatments with a higher impact on plant growth parameters. The increase in total dry weight was up to 92% and 89% for T5 and T7, respectively. These treatments also have a satisfactory influence on root development, with 78% and 53% of fresh weight root over the control (Table 3).
G. diazotrophicus has shown satisfactory results in sugarcane inoculation, increasing the levels of dry matter and production when it was inoculated with other endophytic bacteria, increasing by 21% and 18% for the diameter of stem and total dry matter, respectively (Schultz et al., 2017). In this study, those parameters increased by 9% and 92%, respectively when plants were inoculated with LASFBC 1911. The increases obtained for LASFBC 1911 are also greater than reported by Muthukumarasamy et al. (2006) who obtained an increase of 45% for the fresh weight of roots and 35% for total fresh weight. The inoculation with G. diazotrophicus could also increase the anti-oxidative and osmoprotectant compounds that help plant development (Aguiar et al., 2018), that was not tested in this research.
However, co-inoculation with other endophytic or rhizospheric bacteria has offered more consistent results in almost all parameters evaluated, as observed with T7. This treatment considered the inoculation of three bacteria that could fix nitrogen, solubilize nutrients and/or produce IAA. Oliveira et al. (2002) also demonstrated than the co-inoculation with G. diazotrohpicus, A. amazonense, and Burkholderia sp. could increase plant development.
These results are explained according to a combination of properties of bacteria, and how this could give more complete support for plant development (dos Santos et al., 2017). The inoculation of the bacteria mixtures also evidences that there is not high specificity between these bacteria and the source plants. The bacteria different from G. diazotrophicus were isolated from avocado, pineapple, and sugarcane. With these results, many inoculation tests could be delivered employing bacteria from different sources in a high variety of crops.
Table 2 Growth inhibition of phytopathogenic fungi by isolates of G. diazotrophicus
Bacteria | Fusarium sp. | Alternaria sp. | Roselinia sp. | Lasiodiplodia sp. | Sclerotinia sp. |
LASFB 1573 | 61% | 55% | 32% | 31% | 0% |
LASFB 1574 | 70% | 54% | 40% | 49% | 17% |
LASFB 1740 | 67% | 57% | 26% | 35% | 0% |
LASFBC 461 | 75% | 57% | 27% | 32% | 11% |
LASFBC 1911 | 69% | 54% | 30% | 40% | 17% |
Table 3 Effect of inoculation of G. diazotrophicus on physiological parameters in sugarcane variety Mex 73-523
Bacteria | Plant high (cm) | Diameter (cm) | Foliar area (cm2) | Aerial fresh weight (g) | Aerial dry weight (g) | Roots Long (cm) | Fresh Weight root (g) | Dry Weight root (g) | Total fresh weight (g) | Total dry weight (g) |
Control 1 | 30.44 a | 3.3 ab | 22.88 a | 0.7174 a | 0.0946 a | 15.25 abc | 1.0203 a | 0.1095 a | 1.7397 a | 0.2042 a |
Control 2 | 32.71 ab | 3.3 ab | 25.01 ab | 0.7999 ab | 0.1073 a | 16.62 abc | 1.1368 ab | 0.1117 ab | 1.9368 ab | 0.2190 ab |
T1 | 34.43 abc | 3.1 ab | 29.91 abc | 0.8858 ab | 0.1442 ab | 13.84 a | 1.3530 abc | 0.1332 abc | 2.2388 abc | 0.2773 abc |
T2 | 37.43 bcd | 3.1 a | 35.37 c | 1.0675 bc | 0.1526 ab | 14.50 ab | 1.5773 cd | 0.1634 abc | 2.6449 cd | 0.3160 abc |
T3 | 37.95 bcd | 3.2 ab | 34.81 c | 1.0265 bc | 0.1695 ab | 15.97 abc | 1.4285 bc | 0.1795 bc | 2.4550 bcd | 0.3490 bc |
T4 | 36.21 bcd | 3.2 ab | 30.56 bc | 0.9564 abc | 0.2276 b | 15.64 abc | 1.3636 abc | 0.1371 abc | 2.3197 abc | 0.3647 c |
T5 | 39.18 cd | 3.6 b | 32.41 c | 1.2065 c | 0.1968 ab | 16.94 bc | 1.8155 d | 0.1963 c | 3.0221 d | 0.3930 c |
T6 | 39.55 cd | 3.4 ab | 30.40 bc | 1.0618 bc | 0.1637 ab | 17.20 c | 1.4837 bcd | 0.1786 abc | 2.5455 bcd | 0.3424 abc |
T7 | 41.16 d | 3.5 ab | 35.01 c | 1.2067 c | 0.1857 ab | 16.40 abc | 1.5562 cd | 0.2010 c | 2.7629 cd | 0.3868 c |
T8 | 38.43 cd | 3.3 ab | 29.49 abc | 1.0257 bc | 0.1749 ab | 16.55 bc | 1.4996 bcd | 0.1763 abc | 2.5252 bcd | 0.3512 bc |
Control 1: Water, Control 2: SPA medium, T1: LASFB 1740, T2: LASFB 1573, T3: LASFB 1574, T4: LASFBC 461, T5: LASFBC 1911, T6: LASFB 1740 + LASFB 1643 + LASFB 002, T7: LASFB 1573 + LASFBP 086 + LASFB 009, T8: LASFBC 461 + LASFB 1643 + LASFBP 086.
Conclusions
The bacterium G. diazotrophicus is present in vegetal tissues of sugarcane cultivated in the northern zone of Peru. The isolated and characterized native bacteria show a physiological profile that allows them to be classified as plant growth-promoting bacteria, being possible to infer a beneficial effect in subsequent inoculation field tests.
Acknowledgments
The current project was made possible by the support of Innovate Peru Contract Nº 142-PNICP-PIAP-2015, as part of the Ministry of Production of Peru