Introduction
Potato (Solanum tuberosum L.) is the fourth most consumed crop in the world after wheat, corn, and rice, which are exceeds in terms of nutritional value. Among the potato producing countries, Peru is ranked 19th worldwide, being the main producer in Latin America, producing more than 4.5 million tons of this tuber and involving the participation of approximately 600,000 farmers distributed in 19 regions; being La Libertad, Huánuco, Puno, Junín and Ayacucho regions where ancestral crops are still carried out (Ministerio de Agricultura y Riego, 2016).
Potato cultivation is affected by limiting factors such as low tuber quality, soil impoverishment, indiscriminate use of agrochemicals, as well as the attack of insects and phytopathogenic organisms, resulting in a decrease in productivity and profitability of this tuber (Rico, 2009). On the other hand, due to exposure to various pests and pathogens, potato cultivation is an activity with high use of agrochemicals, substances that are toxic and dangerous for the farmer, the crop itself and the environment (Virmond et al., 2017).
A number of viral, fungal and bacterial agents, as well as nematodes, viroids and oomycetes cause diseases to the potato crop (Bertschinger et al., 2017), highlighting among them, the Oomycete Phytophthora infestans (Mont.) De Bary, causal agent of the disease "late blight", and the main responsible for the deficit in yield and production, being able to cause from slight, moderate damages, until the total destruction of crops (Cardona-Piedrahita et al., 2016). The main sign of late blight is the burning of the foliage and the dry rot of the tubers (Stefańczyk et al., 2016).
Currently, antagonistic microorganisms are used to control fungi and oomycetos in different types of crops, reducing the use of chemical methods that cause a negative environmental impact and induce the emergence of resistance in the future (Marín et al., 2013). Actinomycetes as Streptomyces sp. are capable of synthesizing bioactive compounds that inhibit the development of certain phytopathogens, thus providing protection to crops (Sinha et al., 2014). Some important studies include the control of phytopathogens such as Verticillium dahliae in cotton (Xue et al., 2013), Valsa mali in apple trees (Tang et al., 2015) and Fusarium oxysporum in soybeans (Mariastuti et al., 2018),
Some microorganisms such as Pseudomonas aeruginosa (De Vrieze et al., 2019), Lysobacter capsici (Puopoloet al., 2015), and the fungi Aureobasidium pullulans (Di Francesco et al., 2017) have been previously used in the control of Phytophthora infestans with great results. However, there are not many studies of the use of actinomycetes for the control of this pathogen. In this context, the aim of the present study is to contribute to the current knowledge about certain native strains of rhizospheric actionmycetes that have antagonistic capacity with potential use as biological controllers of Phytophthora infestans that affects potato crops.
Material and methods
Maintenance of Phytophthora infestans
Phytophthora infestans, strain PHU-117, was maintained by subcultures of Rye Agar (HiMedia, India), incubating the plates at 28 °C from 5 to 7 days.
Sampling and transport to the laboratory
Sampling took place in potato fields located in the town of Cabana, Lucanas, Ayacucho (14° 17' 10" South Latitude; 73° 57'51" West Longitude). The rhizospheric soil samples were collected from native potato plants of Canchán, Futis, Huayro, Cuchipa-akan, Ccompis and Chaska varieties, collecting 200g portions of soil in ziploc bags, and then sent to the laboratory for processing
Isolation and selection of actinomycetes
10 g of soil from each dry sample was weighed, placing them inside a sterile bottle with 90 mL of 1.5% phenol saline solution and incubating at 30 °C for 30 minutes. Subsequently, serial dilutions were prepared up to 10-4, sowing 100 µl in plates with Oat Agar (BD DifcoTM, UK), Asparagine Agar (Merck, Germany), Czapeck Agar (Merck, Germany) and Casein-Starch Agar (HiMedia, India), all supplemented with nystatin (50 μg/mL) and nalidixic acid (25 μg/mL) (Sigma-Aldrich, USA), incubating them at 28 °C from 15 to 20 days (Cisneros, 2016).
Morphological characterization of isolated actinomycetes
Actinomycetes were characterized macroscopically by observation of the colonies. The microscopic characterization was performed using microcultures, comparing the characteristics with those described in the Bergey's Manual of Systematic Bacteriology (Whitman et al., 2012). Selected strains were processed according to Colona et al. (2014) for observation by a scanning electron microscopy Inspect TM S50 (FEITM, Japan).
Assimilation of sugars and activity of hydrolytic enzymes
Glucose, sucrose, and mannitol metabolism tests were performed in ISP 9 medium with 1% of carbohydrates (w/v) (Caro, 2016). Likewise, the ability to produce amylase and cellulase were determined in Almidón Agar and Mandels-Reese Agar respectively, being evaluated according to Pérez et al. (2015)) with Lugol and Congo red solutions. In all cases, the tests were evaluated up to 15 days.
Growth depending on pH and temperature
The tests were performed according to Calvo and Zúñiga (2010), using Oat Agar, evaluating its development at pHs 5.5; 7 and 8.5, and the temperatures of 4, 28, 30 and 45 °C, incubating them up to 10 days. The intensity of growth was evaluated at the end of the test period, measuring the diameter of the colonies, and recording the average size.
Actinomycete antagonism against Phytophthora infestans
In vitro antagonism was performed by dual culture (Pérez et al., 2015), sowing a suspension of actinomycete spores (105 CFU/mL-1) in Oat Agar and Rye Agar plates at 3 equidistant sites, being in the center an inoculum of P. infestans. The plates were incubated at 28 °C for 10 days. The diameters of the inhibition halos were measured with a millimeter ruler. The percent inhibition (PI) was calculated according to Ezziyyani et al. (2004): Percent inhibition (PI)=(R-r)/R x 100
Where, R is the major radius (in mm) of the Oomycete colony (control); r is the smallest radius (in mm) of the Oomycete in the dual culture. In all cases the radius measurement was the average of triplicate measurements.
PKS gene detection and molecular identification of actinomycetes
Selected strains which had the best antagonistic results were seeded in fermentation broths and incubated under stirring at 28 oC for 7 days. Then, they were centrifuged at 14,000 rpm for 10 minutes, adding 1 ml of Lysozyme (20 mg/ml) (Sigma- Aldrich, USA) to the pellet, keeping in incubation at 56 °C for 1 h. DNA extraction was performed using GeneJET Genomic kit (Thermo Fisher Scientific, UK). For PCR amplification of PKS genes, primers K1 and M6R were used, whose sequences are 5′-TSAAGTCSAACATCGGBCA-3′ and 5′-CGCAGGTTSCSGTACCAGTA-3′respectively, according to Chen et al. (2019) protocol, whose detection was revealed by agarose electrophoresis by visualization of the band at 700 bp in a transilluminator (BioBase, China). Molecular identification was performed by PCR amplification of 16S rRNA gene using universal primers 27F and 1429R, whose sequences are 5′-AGAGTTTGATCMTGGCTCAG-3′ and 5′-GGTTACCTTGTTACGACTT-3′respectively, following the Parada et al. (2017) protocol. The amplicons were revealed by electrophoresis in agarose gels (1300 bp) and sent to Macrogen Inc. (Korea) for sequencing. The chromatograms were evaluated and edited in MEGA7 program, with multiple alignments of 16S rRNA sequences belonging to other actinomycetes of GenBank database, using Bacillus subtillis as an external group. The phylogenetic tree was constructed using Neighbor-Joining method (Kumar et al., 2016).
Results and discusion
Characterization of isolated actinomycetesTable 1 Figure 1
The microscopic characterization by microculture showed fragmented mycelia and long spore chains. At Gram staining, Gram positive spiral filaments were observed (Figure 1C), typical of the genus Streptomyces. Likewise, the observation by scanning electron microscopy of selected strains allowed distinguishing branched filaments (Figure 2A) and presence of abundant spores (Figure 2B).
Oat Agar is not usual for the study of actinomycetes; however, it was used in this case following the recommendations of Franco-Correa et al. (2010), who isolated important native strains of actinomycetes with potential application of Plant Growth Promoting Rhizobacteria (PGPR) from samples of rhizospheric soil.
Table 1 Macroscopic characterization of actinomycete colonies isolated from the native potato rhizosphere grown
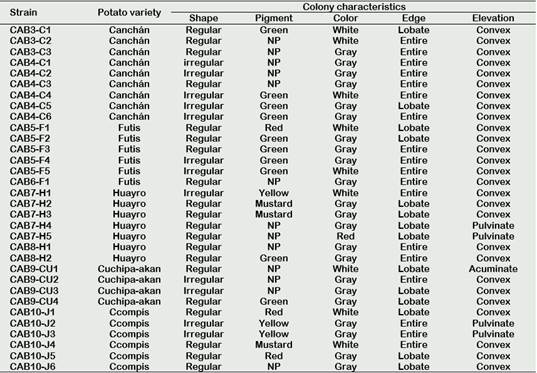
NP: No pigment.
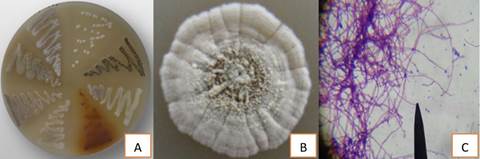
Figure 1 Various colonies of rhizospheric actinomycetes isolated from rhizosphere of native potatoes (A and B); and Gram staining observed under a microscope at 1000X magnification (C).
Sugar metabolism, hydrolytic activity and growth depending on pH and temperature
The results of each test are shown in Table 2. A big number (97%), except for strain CAB7-H5, indicated that they were able to assimilate the three sugars. In contrast, Rico (2009), who also worked with isolated potato actinomycetes, only found that 15% of their isolates could use these sugars. The reason for this difference could be explained by the nature of the samples, since they come from different locations, soil types and physical-chemical factors of each place that influence the growth or activity of microorganisms (Li et al., 2018).
Regarding the ability to produce extracellular enzymes, all strains of actinomycetes showed amylolytic activity (100%); in contrast, only 16 (50%) showed cellulolytic activity. Actinomycetes CAB9-CU4 and CAB10-J2 had the best results of amylolytic and cellulolytic activity, respectively (Figure 3). In this regard, Caro (2016) reports similar results for producers of amylases (89.8%) and cellulases (26.5%).
Also, the actinomycetes showed optimal growth in the pH range from 5.5 to 8.5 and at the incubation temperature of 28 °C, reporting very few strains that could grow at low temperatures (Table 2). Rico (2009) reports that, at a pH 5.5 and a temperature of 10 °C, 65% of its isolates can be developed, although with limitations in their development, presenting colonies of smaller size than at higher temperatures. On the other hand, Cisneros (2016) reports that none of its isolates could develop at acidic pHs and low temperatures. These results indicate that the physical-chemical characteristics of the soil are determinants for the development of actinomycetes, and that they must be taken into consideration when conducting tests in field of biological control of pests.
Table 2 Metabolic activity on carbohydrates, production of extracellular enzymes and growth based on pH and temperature of rhizospheric actinomycetes isolated from rhizosphere of native potatoes
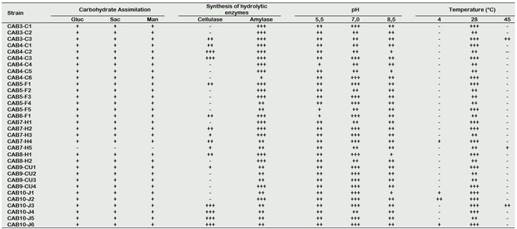
Carbohydrate Assimilation: positive = +; negative = -
Synthesis of hydrolytic enzymes: negative = -; low = +; moderate = ++; good = +++
Growth depending on pH and temperature: negative = -; low = +; moderate = ++; good = +++
Actinomycete antagonist activity against P. infestans
The results of antagonistic activity tests are shown in Table 3. From all isolated actinomycetes (n = 32), 25 (78.1%) showed activity against P. infestans at least in one of the two culture media used here. In Oat Agar, it was possible to observe 25 (78.1%) of strains with positive activity, while in Centeno Agar only 10 (31.25%) had a positive result. The percentages of inhibition obtained varied in the range from 13.33 to 80.05%, being strains CAB10-J2 (80.05%), CAB9-CU4 (77.47%) and CAB5-F5 (37.5%) who pre-sented the highest values of inhibition per-centages (Figure 4).
Table 3 Rhizospheric actinomycetes isolated from native potatoes and their antagonistic activity to Phytophthora infestans in two culture media
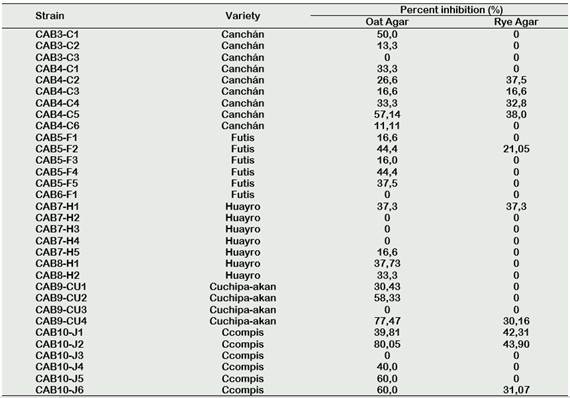
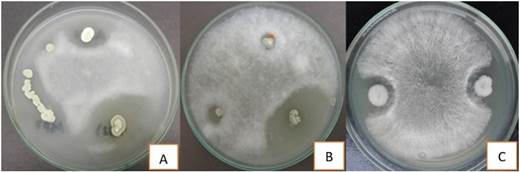
Figure 4 Anti-Phytophthora infestans activity shown by the actinomycetesCAB9-CA4 (A), CAB10-J2 (B) and CAB5-F5 (C).
Performing a comparative analysis, Fonseca et al. (2011) find that, of the total actinomycetes isolated from organic residues of chipaca (Bidens pilosa), only 32% presented antagonistic activity against P. infestans. It should be noted that these tests were performed on Rye Agar. Likewise, Pérez et al. (2015) reported that 42.4% of their isolated actinomycetes from compost showed P. infestans inhibitory activity in Rye Agar. Another similar study was carried out by Caro (2016), indicates that 46.9% of actinomycetes isolated from potato rhizosphere had positive activity against P. infestans in Rye Agar. In the present work, we obtained results of antagonisms superior to those already mentioned; however, it is necessary to specify that these results come from the tests in Oat Agar, which had not been used before for antagonism tests, being a different situation with Rye Agar because it possesses sitosterol, which is necessary for the development of this pathogen (Fonseca et al., 2011).
Some other microorganisms with direct anti-Phytophthora activity have been described. Potential biocontrol candidates for which inhibitory activity against P. infestans was demonstrated include Trichoderma sp., Pythium oligandrum, Bacillus sp. and Pseudomonas sp. (Mariastuti et al., 2018; De Vrieze et al., 2019), which could have in vitro inhibition results superior to those obtained in this study; however, it is necessary to emphasize that in many of them the secondary metabolite that causes the antagonistic effect wasused directly, which is not explored in this study, but it is an important aspect to consider for future researches.
PKS gene detection and molecular identification
Three of the actinomycetes which had the best results were selected for the molecular tests, CAB10-J2, CAB9-CU2 and CAB5-F5, having the 700 bp band of PKS gene (Figure 5).
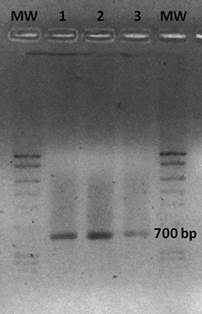
Figure 5 PCR electrophoresis gel indicating PKS gene amplification (700 bp). Lane 1: CAB10-J2. Lane 2: CAB9-CU2. Lane 3: CAB5-F5. MW: Molecular-weight size marker.
These were selected for sequencing of 16S rRNA gen, identifying strains CAB10-J2 and CAB9-CU2 as Streptomyces luteoverticillatus with 99% of similarity, while strain CAB5-F5 was identified as Streptomyces sp. with 99% of similarity (Figure 6). The sequences of these three strains were deposited in the GenBank database (Access numbers: MN416404.1, MN416403.1 and MN4164 12.1).
About S. luteoverticillatus, it is known as a major producer of antifungal compounds like Carbazomycin B (Feng et al., 2019), which would explain the positive antagonistic effect on P. infestans described in this work.
Conclusions
The results of this work provide important data from in vitro tests, although additional studies of Streptomyces and its secondary compounds are required that lead to effective practical formulations for field application. The potential of Streptomyces spp. in the control of plant diseases has been the subject of many studies; on the other hand, reports against P. infestans are scarce. In this sense, our results represent a first step towards the effort to incorporate actinomycetes into biocontrol programs of late potato blight, being and important starting point for the elaboration of bioinoculants of easy elaboration and low cost to increase the yield and reduce the losses by pests of this tuber.