Introduction
The global human population has grown in recent years, and an important increase is projected towards 2050. In consequence, food demand will increase strongly (FAO, 2017). The application of agrochemicals in agriculture is seen as a necessary mana-gement to satisfy the food requirements (Good and Beatty, 2011). The demand for nutrient (N+P2O5+K2O) consumption has increased over the years, reaching 200.522 million MT during 2018 (FAO, 2015). Nevertheless, only 30-50% of nitrogen is taken up by the crops. Consequently, surplus N generates environmental problems (Wang and Li, 2019). Moreover, excess chemical fertilization or fresh manure can have a negative impact on soil properties, altering the soil biota ecosystems, the plantmicroorganism interactions, and water quality. The application of microbial inoculants constitutes a biotechnological tool to improve microbial ecology and plant nutrition, mitigating the negative impact of conventional chemical fertilization.
Microbial inoculants are biological products composed of viable beneficial microorganisms used to improve soil quality and plant nutrient status (Martínez, 2015; Pylak et al., 2019). Pseudomonas, Bacillus, Azospirillum, and Azotobacter are the main genera of benefic bacteria that induce plant growth (Ferreira et al., 2019). Soil-applied micro-organisms can increase plant-growth by nitrogen fixation, phosphate solubilization, exudation of plant hormones, production of siderophores, and production of substances that inhibit plant pathogens (Martínez and Martínez, 2007; Glick, 2012). In particular, bacterial inoculants consortia containing the species Acidovorax facilis, Bacillus licheniformis, B. subtilis, B. oleronius, B. marinus, B. megatherium and Rhodococcus rhodochorus have been evaluated in tomato, pumpkin, corn, and broccoli, among others (Agricen, 2020). The reported beneficial effects of bacterial consortia are, among others, the increase in yield, root biomass, water retention capacity, and nutrient availability (Agricen, 2020). Therefore, the combination of appropriate rates of chemical fertilizer with microbial inoculants can have a significant positive impact on soil quality and crop yield (Bargaz et al., 2018; Lin et al., 2019; Ortega-Blu et al., 2020). Therefore, it is necessary to study the application of chemical fertilizers and microbial inoculants in vegetables of a commercial interest to optimize the fertilization-yield relationship. Based on the above the objective of the present work was to evaluate the impact of a bacterial inoculant and chemical fertilizer, and their combined application on soil properties and nutrition of lettuce (Lactuca sativa L.). It noteworthy pointing out that L. sativa is widely used as a model vegetable in horticulture to evaluate fertilization mana-gement due to its fast germination and growth, and easy management. Additio-nally, L. sativa has a great as it is widely consumed.
Materials and methods
Plant material and experimental design
A greenhouse experiment was performed at the facilities of Federico Santa María University in Santiago, Chile. Plant material corresponded to seedlings of L. sativa L. var crispa L., which were planted on 2.3-L pots in a sandy soil. Selected soil properties are presented in Table 1. The experiment was performed under a completely randomized design. Six treatments were established to evaluate the effects of microbial inoculant application in the presence of chemical fertilization at three levels: 0, 50 and 100% (Table 2). The evaluation was performed for 60 days. The chemical fertilization, at the complete rate, consisted in the application of an equivalent of 60, 50 and 60 hg ha-1 of N, P2O5, and K2O, respectively. Microbial inoculant, consisted in a consortium of the following species: Acidovorax facilis, Bacillus licheniformis, B. subtilis, B. oleronius, B. marinus, B. megatherium and Rhodococcus rhodochorus (concentration 1 x 103 ufc/ml for each). The product was diluted at 1.2% in water for soil application (Agricen, 2020).
Table 1. Chemical characterization of the soil used for the experiment
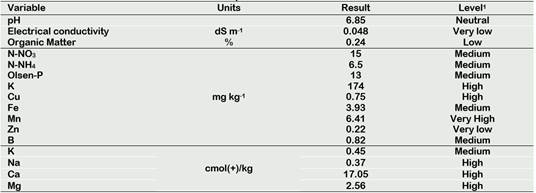
1According with INIA: http://www.inia.cl/link.cgi/suelo/resultados/7706
Table 2. Evaluated Treatments
Treatment | Description |
F100% | Standard chemical fertilization1 |
F100%+SB | Standard chemical fertilization with the application of Microbial Inoculant2 |
F50% | Standard chemical fertilization at a half rate |
F50%+SB | Standard chemical fertilization at a half rate with the application of Microbial Inoculant 2 |
F0% | Control without chemical fertilization |
F0%+SB | Control without chemical fertilization + Microbial Inoculant |
160, 50 y 60 kg ha-1 of N P2O5 y K2O respectively from urea, triple super phosphate and KCl.
2Recommendation of dilution of Microbial Inoculant at 1.2% in water (applied at soil).
Soil response variables
Measured soil variables included available N, extractable P, and enzymatic activity. The quantification of nitrogen and phosphorus available was performed according to Sadzawka et al. (2006). Microbial populations were determined by the plate count (Martínez et al., 2010). The acid phosphatase activity was determined according to the methodology proposed by Tabatatai and Bremmer (1969). Dehydrogenase activity was determined according to Acosta and Paolini (2005). Urease activity was determined using the indophenol blue technique (García et al., 2003).
Nitrogen in leachates
All pots were irrigated with an equivalent amount of water to keep the soil near field capacity. Drained water was collected after each irrigation event from each pot, and stored at 4 °C, before its analysis. Analysis of NO3-N in water was performed using the spectrophotometric method by direct quantification at 220 nm (Gvozdić et al., 2009). On the other hand, the quantification of NH4-N was performed by a colorimetric method (Mulvaney, 1996).
Leaf analysis and nutrient use efficiency
Fresh vegetable tissues were decontaminated through sequential washes with HCl (0.1 M) and sterile distilled water, and then dried at 70 ± 5 °C to determine root and leaf dry mass. Nutrients leaf concentrations were determined by the methods described by Sadzawka et al. (2007). Leaf total chlorophyll content was determined according to Lichtenthaler and Wellburn (1983).
Results and discussion
Sustainable agricultural practices for crop production are needed to ensure food security and environmental protection (McLaughlin and Kinzelbach, 2015). Chemical fertilizers have been widely applied to increase food production. On the other hand, the application of microbial inoculants constitutes a strategy to improve soil quality and plant nutrition (Martínez et al., 2010; Pylak et al., 2019). Recent studies have shown the important effect of the joint application of chemical fertilizer and microbial inoculants (Bargaz et al., 2018). A study performed by Ye et al. (2020) reported that an inoculant containing Trichoderma species and reduced rate of chemical fertilizer increased tomato yield and it improved microbial activity in the soil. Moreover, Assainar et al. (2018) indicated that an adequate combination of microbial inoculants with rock-based fertilizer improved grain yield in maize under conditions of glasshouse. Nevertheless, there is not enough research on the effects of the joint application of both, chemical fertilizer and microbial inoculant on soil quality and plant nutrition. Ortega (2015) described this strategy as integrated nutrient management (INM), which included organic matter application, adjusted chemical fertilization, and microbial inoculants. In particular, Soil Builder®, a commercial microbial inoculant, strongly decreases the emission of nitrous oxide (N2O) (80%) in soils fertilized with urea-ammonium nitrate (Calvo et al., 2013). Afterward, Calvo et al. (2014) reported that Soil Builder® had an important growth-inducing effect, even under vegetables saline stress (i.e Cucurbita pepo) (Calvo et al., 2014).
Natural soil nutrient availability derives from organic matter (OM) mineralization mediated by microbial enzymatic activities, which degrade organic polymers to release nutrients for the root uptake (Dhaliwal et al., 2019). Often, OM mineralization is not enough to support high crop yields, and nutrients needs are supplied by chemical fertilizers. Our results showed that there were no statistically significant differences (p < 0.15) in nitrogen and phosphorus availability among treatments (Table 3). This effect could partly be explained by the high N leaching rates due to the sandy texture of the soil. Nitrogen losses were larger for the F100%+ SB treatment, starting at day15 (Figure 1). The larger N leaching observed in the treatments with microbial inoculant suggested an increase of urease activity.
Table 3 Soil quality indicators after treatment application
Treatment | P-availability (mg kg-1) | N-availability (mg kg-1) | Total bacteria (Log10 UFC g-1) | APA (µg p-nitrophenol g-1 h-1) | Urease Activity (µg NH4 g-1 h-1) | DHA (µg TFP g-1 day-1) |
F100% | 8.3 ± 0.23 | 12.6 ± 4.27 | 5.9 ± 0.2c | 66.0 ± 7.58 | 35.6 ± 4.17ab | 28.3 ± 1.69 |
F100%+SB | 9.8 ± 0.50 | 12.2 ± 0.86 | 6.4 ± 0.2abc | 34.4 ± 2.15 | 49.1 ± 12.43ab | 28.5 ± 1.05 |
F50% | 9.2 ± 0.48 | 3.4 ± 0.43 | 6.2 ± 0.2bc | 29.7 ± 1.71 | 59.1 ± 0.98a | 30.8 ± 1.04 |
F50%+SB | 7.6 ± 0.23 | 9.7 ± 3.19 | 6.3 ± 0.3abc | 40.1 ± 0.84 | 55.4 ± 3.67ab | 28.7 ± 0.67 |
F0% | 11.5 ± 0.31 | 12.1 ± 3.92 | 7.1 ± 0.1a | 40.7 ± 2.69 | 34.8 ± 4.85ab | 27.5 ± 0.97 |
F0%+SB | 20.7 ± 5.19 | 11.1 ± 3.60 | 7.1 ± 0.1a | 44.6 ± 3.06 | 22.8 ± 1.69b | 24.7 ± 0.84 |
p value | 0.317 | 0.147 | 0.002 | 0.043 | 0.040 | 0.209 |
Abbreviations: APA: Acid phosphatase activity; DHA: Dehydrogenase activity.
Total bacterial population was higher in the treatments with no chemical fertilization in the presence or absence of microbial inoculant (Table 3). The inoculation effect was not detected by this variable, maybe because of the low concentration of each bacteria species in the product, 1x103 ufc/ml (Agricen, 2020).
Microorganisms release a variety of enzymes that act on soil organic matter in creasing nutrient availability (Jacoby et al., 2017; Martínez et al., 2010). Phosphatase activity is an indicator of P mineralization of soil. On the other hand, urease activity is an indicator of N bioavailability from organic sources such as urea and their precursors. Furthermore, dehydrogenase activity is an indicator of global soil metabolism. The results revealed that there were no significant differences in terms of enzymatic activities among treatments, which can be explained by the low organic matter content. Microorganisms need a source of energy (carbon) to properly develop and function in the soil. However, it was noted that urease activity tended to be higher in treatments with urea application. These results confirm the importance of adequate organic matter concentration together with the application of microbial inoculants (Martínez et al., 2010; Martínez, 2015).
Leaf nutrient content is an index of nutrient absorption and its availability on soil (FAO, 2006). The results indicated that treatment with microbial inoculant and high fertilization rate significantly increased absorbed N by plants (46.82 mg pot-1), which probably were associated with the urea mineralization capacity of the microbial inoculants (Figure 2a). A similar tendency was found for the P and K absorption, although the effect of the inoculant was rather poor, probably because of the lack of sufficient organic matter to be mineralized by microorganisms (Figure 2bc). Besides, we analyzed the chlorophyll, which is a fundamental molecule to the photosynthesis process. Interestingly, the treatment without fertilization reached the largest chlorophyll content (899.4 μg g-1of chlorophyll A and 169.0 μg g-1 of chlorophyll B), probably because of a concentration effect (Figure 2d). The yield of a crop is measured by the dry mass of the economically important organ harvested (i.e aerial biomass in lettuce) (Figure 2e). Besides, root dry matter is an indicator of the plant capacity for nutrient absorption. Results showed significant differences among the fertilization levels but not between the presence or absence of the inoculant (Figure 2f). The nutrient use efficiency is given by the nutrient availability and root density.
This study demonstrated that the use of a bacteria consortia significantly increased the N (p < 0.001), P (p < 0.002) and K (p < 0.001) use efficiency, reaching 55, 28, and 267% (Figure 3ac). The absorption efficiency indicated that added micro-organisms in conjunction with chemical fertilizer promote improved nutrient use efficiency, usually associated to larger root density (Ortega and Fernandez, 2007; Poblete et al., 2013; Martínez et al., 2010). In our experiment we were unable of detecting changes in root density, probably because of the method used.
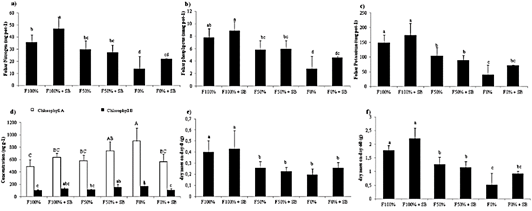
Figure 2 Leaf analysis of L. sativa after treatments application. The a) nitrogen, b) phosphorus and c) potassium absorbed. d) Chlorophyll A and B concentration. e-f). Root dry matter on day-0 and 60 (p < 0.001).
Conclusions
Diverse studies have reported the important role of microbial inoculants to increase soil quality and nutrient use efficiency. The results of the present investigation showed that chemical fertilization treatments had a strong effect on the absorption of nutrients. Microbial inoculant significantly improved the nutrient use efficiency, despite the non-increase in lettuce dry matter. The low microbial concentration in the product and/or low organic matter content in the soil, probably were the limiting factors controlling microorganism’s and metabolites activity in the soil. The increase of available N by the simultaneous application of chemical fertilizer and inoculant was detected only in the leachates. Future studies must be carried out to determine the impact of these treatments on soil quality and productivity in the long and short-term during the growth cycle of lettuce.