1. Introduction
Rice (Oryza sativa) as cereal grain is the most widely staple food consumed for over fifty percent of the world´s population (Lange et al., 2019). It is composed basically of carbohydrates, fiber, proteins, fat and nutritional components like minerals, trace elements, and vitamins, mainly found in brown rice (Sumczynski et al., 2018). However, can also contain various toxic elements (such as As, Pb, Cd, and others) influenced by soil composition, crop management, environment, and genetic factors (Gi et al., 2018; Lange et al., 2019). Among toxic elements, arsenic has been listed as a human carcinogen element by the International Agency for Research on Cancer (IARC) since 1980. The total arsenic content is constituted by both organic and inorganic species. The arsenic toxicity is according to its chemical form, being inorganic forms arsenite As3+ and arsenate As5+, classified in Group 1, the most toxic for human health (especially carcinogenicity) than organic forms. The monomethylarsonic acid (MMA) and dimethylarsinic acid (DMA) are the two main organic form of arsenic, are less toxic, but have been classified in Group 2B as possible cancer promoters.
Rice naturally accumulates higher levels of As, but compared to other staple food crops, rice can absorb and accumulates higher concentrations of As. Besides, as rice is cultivated in flooded soils, anaerobic conditions together excessive water facilitates As mobilization and solubility (mainly arsenite3+), which is absorbed by rice roots, reaching the grains (Mao et al., 2019). Pollution of rice by As has several sources: pollution of paddy soils due to mineral extraction and industrial waste, irrigation of paddy soils with water or groundwater contaminated by arsenic, and indiscriminate use of agrochemicals containing organoarsenic compounds. Drinking water polluted by as is a global concern due to its link to numerous diseases. Likewise, WHO reported that at least 140 million people in 50 countries have been drinking water contaminated by arsenic at level up guideline value of 10 µg/L. To protect health humans from consuming rice contaminated by As, the Joint Food and Agriculture Organization and the World Health Organization (FAO/WHO) recommended that the daily arsenic intake is 0.15 µg/kg. Likewise, the European Commission, through the Regulation (UE) 2015/2016, established advisory levels of 0.2 µg/kg of inorganic As for polished and white rice (European Commission, 2015).
Peru is the world´s third-largest producer of cereal crops and their per capita average consumption is 60 kg/year (Luxbacher & Nolte, 2018). Rice is cultivated mainly in two regions: Selva (San Martin, Amazonas, Cajamarca, Loreto, Huánuco and Ucayali,), and coast region (Piura, Lambayeque, La Libertad, Ancash, Arequipa, and Tumbes). Besides, among the most important pesticides produced and used in Peru are the arsenate, calcium, lead arsenate, and copper sulfate (Mullins, 1965).
However, little or nothing is known about arsenic levels in Peruvian rice samples and the contribution of this staple food to the daily arsenic intake in this South American country. Therefore, the objectives of this study were: i) to quantify for the first time total arsenic (tAs) in 31 rice samples (white rice, brown rice, and parboiled rice) purchased in supermarkets from Luriganco-Chosica, Peru; ii) to estimate the dietary exposure (DE) in the Peruvian population, and iii) to assess the corresponding health risks to the population using the margin of exposure (MOE) method.
2. Materials and methods
2.1. Sample collection and sample classification
Between September and October 2019, one-kilogram bags of 31 different brands of domestic rice produced in Peru were purchased in supermarkets from Lurigancho - Chosica and the metropolitan region of Lima-Peru. The domestic rice samples were divided into subgroups as follows: white rice (n=19), brown rice (n=7), and parboiled rice (n=5). In Peru, rice is traditionally sold in 50-kilogram sacks, but the expansion of supermarket chains, consumer habits are changing towards prepackaged, one-kilogram bags. Rice consumption is expected to increase slightly in MY 2017/2018 to 2.45 MMT and is forecasted to remain constant. Peruvians primarily consume long grain rice (Luxbacher & Nolte, 2018).
2.2. Reagents and materials
Ultrapure water (resistivity 18.2 MΩ cm) was used and obtained from Milli-Q water (Milli-Q water purifier system, Millipore Corp., Bedford, USA). Nitric acid (HNO3) 65% P.A. (ISOFAR, Duque de Caxias, Brazil) was purified and sub-distilled before use for the solution and sample preparation.
2.3 Sample preparation and measurement
100 g of each sample was dried at 60 ◦C using an oven, ground with mortar and pestle, and placed into fifty milliliters of Sterile Falcon® conical tubes before digestion analysis. Between each sample, mortar and pestle were washed with deionized water and dried in an oven, respectively. For digestion, the 3050B EPA (US Environmental Protection Agency) method was adopted. Then, 500 mg of each sample (in triplicate) were weighted accurately in 50 mL canonical tubes (Falcon tubes, Sarstedt, Brazil), added 2.5 mL of sub-distilled HNO3, and closed for predigested for 24 h. After that, all samples were placed on a hot plate at ~ 90 ◦C for 5 hours. During this period, the cover of each sample was opened slightly from time to time to allow that internal pressure caused by gases (nitrous gases and carbon dioxide) be released. After that, the digests were left to cool and diluted to 25 mL using 5% HNO3.
Total As (tAs) content of the rice powdered samples digested, and diluted were quantified by inductively coupled plasma-mass spectrometry (ICP-MS, NexION 300X, PerkinElmer-Sciex, USA) employing the dynamic reaction cell system (DRC-ICP-MS). Likewise, other elements (Al, Ba, Ca, Cd, Co, Cr, Cu, Fe, K, Mg, Mn, Mo, Ni, Na, Pb, Rb, Sb, V, and Zn) and As without use, the DRC system were also quantified by ICP-MS. For this, a calibration curve of six points using Rhodium (20 ppb) as an internal standard for ICP-MS was prepared. To check the accuracy of digestion and analytical procedures, the certified reference material IMEP-19 “trace elements in rice” was used.
2.4. Estimation of the dietary exposure (DE) and margin of exposure (MOE) of As from rice consumption by Peruvians
Dietary arsenic exposure was assessed using the method recommended by FAO/WHO (FAO/WHO, 2005) through the following equation:
where DE (μg/kg bw/day) is the dietary exposure arsenic intake of the studied population; Xk is the daily intake of rice; CAs is the mean concentration of tAs in rice grain, and BW is the average body weight. Total annual per capita rice consumptions of 60.0 kg person/year was obtained from Peru Grain and feed annual report (USDA, 2019). Using this information, the consumption daily of rice by Peruvians was 0.1644 kg/person per day. The average body weight (BW) of the Peruvian population was 60.10 kg, obtained considering the age (teenagers to seniors (aged 14 to 85 years old)) and gender (male (56.77 kg) and female (BW=56.77 kg)) information provided by Health Ministry (MINSA, 2011). For the weekly intake of As the DE was multiplied by seven. Likewise, DI, DE, were estimated assuming that 80% of the tAs are considered as inorganic arsenic (iAs) as reported by Otero et al. (2016).
The human health risk of dietary exposure was evaluated computing the margin of exposure (MOE). The MOE provides a possible assessment to determine the dangerousness of substances and is defined as the ratio of benchmark dose lower bound (BMDL) on estimated dietary exposure. In this work, the BMDL0.5 estimate of 3.0 µg/kg BW/day prescribed by JECFA (Gundert et al., 2015; Jallad, 2019) was used. A MOE of less than 1 indicates a high health risk, whereas a MOE of greater than 1 indicates an acceptably low risk (EFSA, 2005).
2.5 Statistical analysis
One-way ANOVA was used to compare tAs concentrations among different rice groups and a subsequent post hoc Tukey test was applied to determine significant differences. The levels were considered when p ˂ 0.05. Shapiro-Wilk test confirmed the normality of the data. Kolmogorov-Smirnov. Statistical analyses were all performed in the CRAN R version 3.2.6 free software (R Team Core, 2019), using the ggplot2 (Wickham & Chang, 2016) and multicomp (Hothorn et al., 2020) packages.
3. Results and discussion
3.1 As concentration in rice
The tAs quantification in this work was performed on 31 domestic rice samples purchased in supermarkets. The concentrations ranged from 0.163 mg kg-1 to 0.577 mg kg-1, with a mean of 0.308 ± 0.108 mg kg-1. From total samples, 19 were of white rice (mean ± standard deviation (S.D.); 0.292 ± 0.106 mg kg-1), 07 were of brown rice (0.401 ± 0.08 mg kg-1), and 05 were of parboiled rice (0.229 ± 0.03 mg kg-1). A significant difference (p < 0.05) was found among the mean tAs for parboiled and white rice with brown rice (Figure 1).
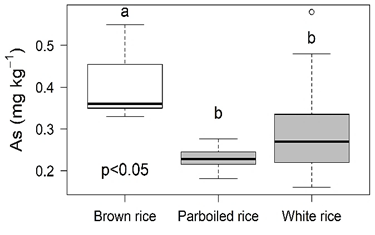
Figure 1. Boxplot of total arsenic (tAs) in brown rice (n=7), parboiled rice (n=5), and white rice (n=19). (line=mean, box = 25th and 75th percentile). Different letters indicate significant differences (p ˂ 0.05).
In the Figure 2 is shown the tAs levels measured in each sample and every type of rice compared to the maximum limits of tAs set by Mercosul (0.3 mg kg-1) and European Commission (0.25 mg kg-1). From the 31 samples, 12 (38.7%) and 17 (54.8%) rice samples were above the limits of Mercosul and European Commission, respectively. Among the 19 samples of white rice, 6 (31.58%) were above the maximum limit prescribed for Mercosul and 1 (63.16%) was above the European limits, respectively. All samples from brown exceeding the limits prescribed by Mercosul and the European Commission. Most parboiled rice samples were lower than both Mercosul and European Limits.
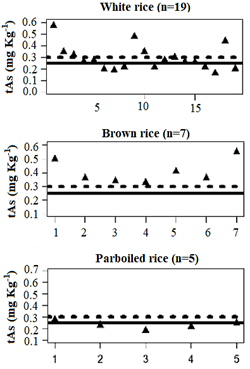
Figure 2. tAs (total arsenic) concentration (mg kg-1) in rice samples separated by type and compared to the maximum limits prescribed by European legislation (0.25 mg kg-1; straight line) and Mercosul (0.3 mg kg-1; dotted line).
The tAs determination in rice may be used as a previous screening to evaluate this element. However, if levels of tAs are above the maximum limit (ML) of 0.2 mg kg-1, as recommended by the Codex Alimentarius Commission of the Food and Agriculture Organization (FAO/WHO CODEX). From Figure 3 is noted that most (n= of the tAs quantified in all samples and types of domestic rice were above the maximum level prescribed by FAO/CODEX (0.20 mg kg-1). According to our results, speciation should have been carried out. However, due to financial support, and lack of infrastructure it was not carried out.
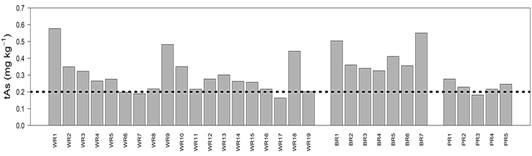
Figure 3. tAs (total arsenic) concentration (mg kg-1) for each sample of rice compared to the maximum level (ML; 0.20 mg kg-1) which speciation analysis is prescribed by Codex Committee on Contamination in Foods (FAO/CODEX).
Table 1. tAs (total arsenic) and concentration of other elements measured in domestic rice grains: white rice (n=19), brown rice (n=7), and parboiled rice (n=5) collected from supermarkets in Peru
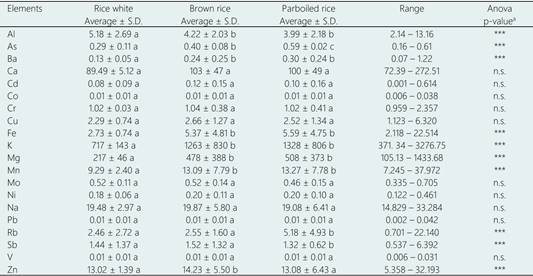
***Significant at 0.05 probability level; n.s.: no significant difference; aValues on each line horizontal line followed by the same letter do not differ significantly (p ˃ 0.05).
Table 2. Concentration of tAs in mg kg-1 in commercial rice grain from different countries
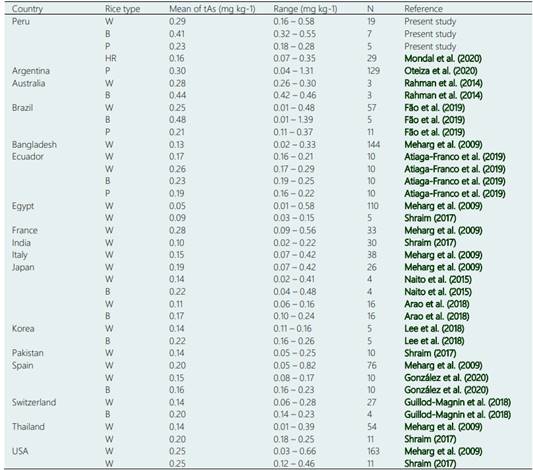
P= parboiled; N = number of samples; W = white rice; B = brown rice.
The average ± standard deviation, range, one-way ANOVA, and Tukey test for tAs and other twenty trace elements are presented in Table 1. The mean concentrations of trace elements in rice samples decreasing in the following order: K ˃ Mg ˃ Ca ˃ Na ˃ Zn ˃ Mn ˃ Al ˃ Fe ˃ Rb ˃ Cu ˃ Sb ˃ Cr ˃ Mo ˃ As ˃ Ni ˃ Ba ˃ Cd ˃ Co, Pb and V. Significant differences were observed in rice grains were found for Al, As, Ba, Fe, K, Mg, Rb, Sb, and Zn. Zinc, Mg, Mn, Cu, Fe, and Co are essential elements and have many beneficial effects on the human body (Shraim, 2017). However, the presence of toxic elements affects adversely the accumulation and transportation of essential elements in plants producing alterations in their morphological parameters, reduction in root length, suppressed seed germination, and reducing the photosynthetic rate (Arif et al., 2019). As rice cultivation is irrigated using water contaminated with toxic metals (As, Cd, and Pb) in Peru, measurement of toxic elements (e.g., Cd, Cr, Ni, Pb, and Sb) is highly recommended for applying for better public health protection and regularly monitor not only arsenic in rice grain (Shraim, 2017).
3.2 Comparison of tAs concentration in domestic rice from Peru with studies from other countries
Table 2 shows a comparison among tAs concentrations in domestic rice (white and brown rice) found in this study and the tAs reported in other studies carried out in different countries. For white rice, when compared with other countries of all world, the average tAs in 19 rice samples 0.292 ± 0.106 mg kg-1 (range 0.163 to 0.577 mg kg-1) was higher than reported in all countries, but similar tAs from Australia, Brazil, Ecuador, France, and USA; while the lower concentration of tAs was reported in Bangladesh, Ecuador, Egypt, India, Italy, Japan, Korea, Spain, Switzerland, and Thailand. For brown rice, the average tAs of 07 rice samples 0.406 ± 0.08 mg kg-1 (range 0.326 - 0.550 mg kg-1) was higher than tAS concentration reported in rice from Ecuador, Japan, Korea, Spain, and Switzerland, but lower tAs concentration than Australia and Brazil.
3.3 Estimation of daily intake (EDI) and margin of exposure (MOE) of from domestic rice grain consumption by Peruvians
Table 3 summarized the results estimated obtained by calculating dietary intake (DItAs, DIiAs), dietary exposure (DEtAs, DEtAs), and margin of exposure (MOE) following the approach previously mentioned in materials and methods. From table 3 is observed that a Peruvian consumes 5.60 μg tAs kg-1 BW weekly. Among the types of rice, brown rice showed the highest DI and DEA values. Furthermore, Table 3 shows the MOE values for all types and concentrations of As in the Peruvian population ranged from 2.25 to 5.87 for tAs and from 2.81 to 7.34 for iAs.
This study report for the first time tAs concentrations quantified in several commercial brands of white rice and brown rice grain purchased in different Peruvian markets. Likewise, was compared the tAs concentration measured with the tAs maximum limits set by Mercosul (0.3 mg kg-1), European Commission (0.25 mg kg-1), and FAO/CODEX (0.20 mg kg-1). Most of the rice samples exceeding the tAs values proposed by regulatory agencies.
Table 3. Estimated dietary intake (EDI), dietary exposure (DE), and margin of exposure (MOE) computed to tAs an iAs (assuming that 80% of tAs according to Otero et al. (2016))
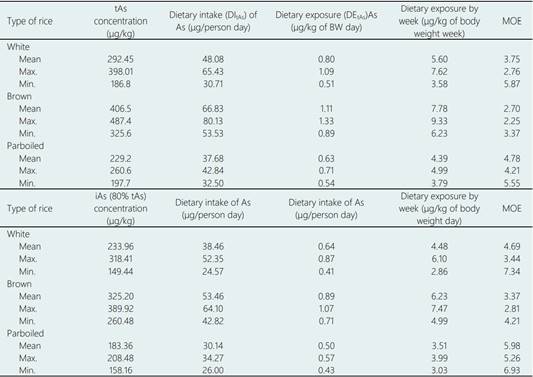
Rice compared to other grains can absorb and accumulates As from soil and water more readily (Zhao & Wang, 2020). Likewise, irrigates agricultural fields with arsenic-contaminated water produce accumulation of As in agricultural soil, and subsequently, As concentration is transferred and accumulated in crops (Mitra et al., 2017). For instance, in Peru, George et al. (2014) reported the presence of As in 116 (77%) samples of water that exceeding WHO recommended limit (10 μg/L) a total of 151 water samples (from groundwater and surface water) collected from 12 districts of Peru. The presence of arsenic in the environment is also related to geological factors (Mexico, Argentina, Chile, and Peru), human activities involving mainly mining and refining of metals (Chile, Bolivia, and Peru), and the use of pesticides during agricultural activities (Quinteros et al., 2017). Mining is a key part of the Peruvian economy, but impacts on water quality, soil, and health human is a problem that has been expanding for almost half a century. The WHO reported that the general population in Peru is exposed to higher levels of lead and arsenic recommended as a consequence of mining activities (George et al., 2014). Besides, Peru together with China, France, and other countries were considered as the main global producer of arsenic for insecticide and pesticide production (Mandal & Susuki, 2002). Likewise, Bech et al. (1997) reported contamination of soils and plants for arsenic and other elements around a copper mine in the Andes of Northern Peru, where rice in main cultivated. Therefore, the presence of arsenic in Peruvian rice may be related to both mining activity and the use of pesticides on agricultural activities (De La Cruz et al., 2018).
Brown rice showed higher tAs levels than white rice. For brown rice, similar results (brown rice = 0.189 mg kg-1 and white rice = 0.132 mg kg-1) were reported by (González et al., 2020) in Spain; (Lee et al., 2018) in Korea (brown rice = 0.22 mg kg-1 and white rice = 0.14 mg kg-1), and (Rahman et al., 2014) in Australia (brown rice = 0.44 mg kg-1 and white rice = 0.28 mg kg-1). Likewise, in the scientific literature is reported that brown rice present 80% more iAs than white rice (Hassan et al., 2017). Corroborating our results, several authors reported higher tAs concentration levels in brown rice than white rice (Atiaga-Franco et al., 2019; Batista et al., 2011; Fão et al., 2019). Lower tAs concentration in white rice compared to brown rice is related to the removal of the outer layer (bran layer; more high levels of As are concentrated here) of the rice grain, during the polishing process to obtain white rice (Atiaga-Franco et al., 2019). Total arsenic in parboiled rice ranged of 0.158 to 0.208 mg/kg. These results showed a slighter lower tAs concentration in parboiled than non-parboiled rice (p˃0.05). A similar result was reported by Fão et al. (2019) in Brazil and Atiaga-Franco et al. (2019) in Ecuador. For reduces As concentration in rice grain previously consumption, several authors recommended washing, soaking, and precooked previously the rice (González et al., 2020; Naito et al., 2015).
The Joint FAO/WHO Expert Committee on Food Additives (JEFCA) set the provisional tolerable daily intake (PTDI) of 2.1 μg/kg body weight per day. In this work, tAs dietary exposure obtained (0.80 μg/kg body weight per day) did not exceed these set values. Likewise, the DEtAs can be considered sure considering the European Food Safety Authority (range of iAs exposure of 0.3 μg/kg body weight per day) based on 0.5% increased incidence in lung, skin, and bladder cancer. However, further studies to elucidate the risk perception of As exposure from rice intake are needed.
The MOE value is recognized as an essential parameter to assess the health risk associated to the consumption of food contaminated by substances that are both genotoxic and carcinogenic (EFSA, 2005). Based on the MOE criteria, Peruvians have a low risk. However, arsenic problem in Peru requires more research and attention to know the actual situation and propose suitable alternatives to reduce As content in rice grain and ensure food safety and human health.
4. Conclusions
Thirty-one samples from the main domestic rice expended in the different supermarkets from Lurigancho-Chosica, Lima-Peru, were evaluated. Although As speciation was not carried out, results showed that there were rice samples in Peru exceeding the maximum limits prescribed by Mercosul, European legislation, and FAO/WHO. However, more investigation is needed. The estimated dietary intake was below the values established by JECFA. MOE value showed value higher than 1, but the possibility of a risk cannot be excluded to some consumers. The results obtained in this work provide important data about tAs content in domestic rice grain expended Peruvian rice, contributing to the lack of knowledge about this pollutant in rice from South American countries.