1. Introduction
Starches are a versatile and widely used material, with undeniable economic importance. They are composed mainly of two polysaccharides, amylose, and amylopectin, that are organized in complex structures called granules. Different sources present different molecular and granular characteristics; consequently, different properties are available depending on the starch source and processing conditions. The main characteristics of the starch structure are well established in the literature and can be found elsewhere (Eliasson & Gudmundsson, 2006; French, 1984; Hizukuri et al., 2006; Liu, 2005; Pérez et al., 2009).
Starch gelatinization is one of the most explored phenomena in starch science and has been explored in both classical (Cooke & Gidley, 1992; Hari et al., 1989; Jane et al., 1999; Lund & Lorenz, 1984; Tester & Morrison, 1990) and current literature (Carlstedt et al., 2015; Huang et al., 2017; Tao et al., 2018). In short, the gelatinization process occurs when propitious conditions are present, as sufficient amount of water and temperatures higher than the gelatinization temperature of the given starch. In those conditions, the intermolecular bonds that maintain the crystalline structure of the granules are weakened, and the water in the vicinity can penetrate the formerly rigid granular structure. At this stage, the starch granule swells and loses its birefringence (Xie et al., 2006). The amount of water required, as well as the initial gelatinization temperature, vary according to the starch source, which means that there is no single and ideal gelatinization condition for all starches. Therefore, each starch source demands individual studies, in order to characterize their gelatinization conditions and then attain the best performance.
Furthermore, despite the massive amount of works on this subject, and despite the different techniques employed aiming to better characterize the gelatinization behaviour of different starch sources, this phenomenon can still be unpredictable in different conditions. An evidence of this unpredictability is the raising of recent articles whose purpose is to model the gelatinization stages of different starches (Anuntagool et al., 2017; Desam et al., 2020; Karakelle et al., 2020; Palabiyik et al., 2017; Xu et al., 2020), which proves that it is a still unsolved problem.
Meantime, the importance to understand the gelatinization process and how it affects the starch properties goes beyond the scientific approach. The functional properties of different starchy food products, as their texture and digestibility, are closely related to the gelatinization and retrogradation processes. Consequently, most of the industrial applications involve gelatinized starches or their gelatinization (Avérous & Halley, 2014; Jobling, 2004).
Although there are several ways to evaluate the rheological behaviour of starchy dispersions and solutions, their evaluation using a Rapid Visco Analyser (RVA) is one of the most popular. Furthermore, the majority of this tests are performed using the RVA standard protocols of analysis, such as standard RVA slurry concentration (usually fixed at 10.7% m/m, corrected to 14% moisture in wet basis) and standard RVA temperature profile (50 °C - 95 °C - 50 °C) (Balet et al., 2019).
It is comprehensible that it is necessary to standardize the analysis conditions to ensure a fair comparison between different samples. This occurs since the behaviour of each starch source under different process conditions is difficult to precisely determine, since it is influenced by several factors, as temperature rate, starch concentration and shear forces (Anuntagool et al., 2017). Furthermore, different starch sources imply in different particles size, granules morphology, molecular weight, and amylose/amylopectin ratios, which completely changes the gelatinization behaviour of the samples (Jane et al., 1999; Martins et al., 2021).
However, the standard protocols of starch analysis may not represent the conditions under which they are applied in the industry. Therefore, the evaluation at different conditions may be important to understand from a more realistic approach the starch behaviour, also to propose other possible applications.
In this work, the effect of four starch concentrations from different sources (cassava, maize, high-amylose maize, waxy maize and potato) and two initial and final RVA temperatures were evaluated. For this purpose, the pasting properties (using an RVA equipment), the gel strength (using a texture analyser) and the inherent characteristics of the starch samples were evaluated and compared.
2. Materials and methods
MaterialThe potato (Solanum tuberosum L., Monalisa cultivar) starch sample (presenting ~28% of apparent amylose) was extracted and characterized according to the described in Castanha et al. (2017). The maize starch (~28% of amylose, Argo CS 3400), high-amylose maize starch (~72% of amylose, Hylon VII), waxy maize starch (~98% of amylopectin, Amisol 4000) and cassava starch (~20% of amylose) were donated by “Ingredion Brasil Ingredientes Ltda” (São Paulo, Brazil). All the starch samples presented a purity higher than 99%.
Starch evaluation
Granules morphology: light microscopy and Particle Size Distribution (PSD)
Images of the starch granules were obtained through a light microscope (model L1000 with a 20 W halogen lamp, Bioval, Curitiba, Brazil). The samples were prepared as described by Castanha et al. (2017), 0.1 g of each starch were dispersed in 10 mL of distillate water with 50 μL of Lugol. Then, a drop of this dispersion was observed at room temperature. The magnification of 400x was used and a portable camera of 1.3 megapixels was used to register representative images.
The Particle Size Distribution (PSD) of the starch granules was determined using a Laser Analyser (Partica LA-950V2 Laser Particle Size Analyser HORIBA, Japan), according to the described by Castanha et al. (2018), using ethanol (99.5%) to disperse the samples. The software LA-950 for Windows (HORIBA, Japan) was used to calculate the distribution of the particles, obtained through the volume-based mean diameter.
Pasting properties
The starch pasting properties were determined using a Rapid Visco Analyser (RVA, model RVA-4, Newport Scientific Pvt. Ltd., Australia, with the Thermocline for Windows software version 3.0). Different starch/distilled water proportions were used: 1 g /27 g (3.6%), 2 g /26 g (7.1%), 3 g /25 g (10.7%, being this concentration used in the standard protocols of the RVA equipment) (AACC, 1999) and 4 g /24 g (14.3%). The starch mass was always corrected to 14% moisture in wet basis.
Further, two different temperature combinations were used in this work, aiming to evaluate the effect of the initial and final temperatures on the starch gelatinization process. In the first combination, the suspensions were first held at 50 °C for 1 min, then heated until 95 °C during 7 min and 30 s, being then kept at this temperature for 5 min, followed by cooling to 50 °C for 7 min and 30 s, and finally hold at 50 °C for 2 min. This first combination is the standard protocol of the RVA equipment. In the second combination, the initial and final temperatures of the RVA protocol were lowered from 50 °C to 40 °C maintaining the times used in the first combination.
Gel strength
The starch gel strength was evaluated through a puncture test using a Texture Analyser (TA.XT Plus, Stable Micro Systems Ltd., Surrey, UK; load cell of 50 kgf/ 490.3 N) according to described by Castanha et al. (2017). For this, the paste obtained after the RVA tests were transferred to plastic cups (40 x 20 mm, diameter x height) and stored at 5 ± 2 °C for 24 h for gelification. The cups were kept inside a desiccator with water to ensure uniform moisture distribution. After that, the penetration test was performed in the obtained gels by using 0.5 inches (1.27 mm) diameter cylindrical probe (P/0.5R) at 1 mm∙s-1 until the distance of 15 mm. The force measured by the equipment as a function of the penetration depth was plotted, and the strength of the samples was evaluated through the total energy required for the penetration (which was obtained through the area below the “force versus penetration” curve).
Statistical analysis, Hierarchical cluster analysis (HCA) and Principal Components and Classification Analyses
Each test was performed at least in duplicate for each starch sample. When relevant, a statistical analysis was performed on the treatments through analysis of variance (ANOVA) and Tukey’s test, using the Origin software, version 9.1 (Microcal Inc., Northampton, MA, USA). A significance level of 5% was considered.
A Hierarchical Cluster Analysis was performed to classify the studied samples according to their main parameters of the pasting properties similarities of each starch source under different analysis conditions (concentration and temperature). The used Amalgamation rule was the “Single linkage”. In addition, Principal Components Analysis was performed to evaluate which and how the evaluated factors (RVA parameters, Volume, Area, and amylose content) correlate with the starch source, concentration and temperature. Statistica 12.0 (StatSoft) software was used for both analyses.
3. Results and discussion
Morphology and size distribution of starch granulesThe morphology and size distribution (PSD) of the granules of the different starch samples are illustrated in Figure 1. The shape and size of the granules is characteristic of each source, being the potato starch granules the ones that present a more defined morphology than the other sources. Starch granules from potato are described as ellipsoids, the normal corn and cassava starch granules as polyhedral with the difference that cassava starch granules are more round in shape (Mishra & Rai, 2006). Regarding the granules of the different corn sources, it was reported that the shape is more regular for high-amylopectin starches compared to high-amylose starches which are smother than the others (Chen et al., 2006). Regarding the PSD analysis, the behaviour of the curves indicated how dispersed the granule sizes are in each sample. In addition, the PSD allow to obtain the mean diameter of starch samples, which can be ordered as follows: High amylose maize (diameter = 15.2 µm) < maize (17.4 µm) < cassava (22.8 µm) < waxy maize (26.1 µm) < potato (39.2 µm). These measurements were calculated based on the volume-based mean diameter (D[3,4]) (Bengtsson & Tornberg, 2011; Lopez-Sanchez et al., 2011). This trend in sizes was reported by for potato, cassava and maize starches (Aoyagi et al., 2021;Mishra & Rai, 2006), and the different types of corn starch sources (Chen et al., 2006), where the high amylose starch has the smallest size.
Pasting properties
The pasting properties behaviour of all starch samples using two initial and final temperatures (40 versus 50 °C) are presented in Figure 2. By analysing the effect of the two temperatures in the same starch source at the same concentration, the samples did not present a significant effect (p > 0.05) in most of their pasting parameters (it was evidenced similar pasting behaviour for both temperatures in Figure 2). Therefore, the temperature effect was not deeply explored in this work, and only the main parameters obtained from the pasting properties using RVA at standard temperature protocol (50 °C) (Figure 3) were presented in Table 1. It is important to mention, however, that the RVA profiles (Figure 2,3 andTable 1) were distinct and characteristic for each starch source and concentration.
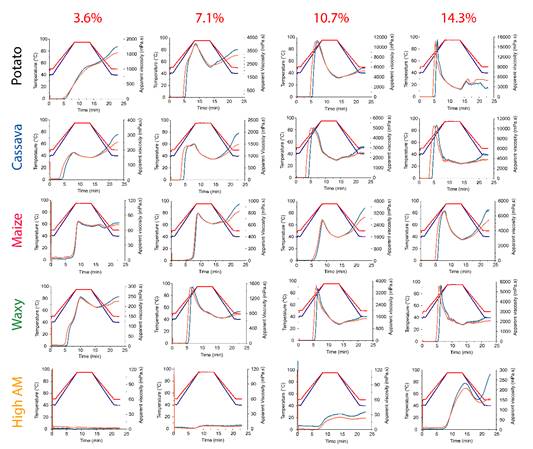
Figure 2 Pasting properties by using the RVA with the tests starting and finishing at 40 °C (blue lines) and 50 °C (red lines). From top to bottom: potato, cassava, maize, waxy maize, and high amylose maize starches. From left to right: starch concentrations at 3.6%, 7.1%, 10.7% and 14.3%.
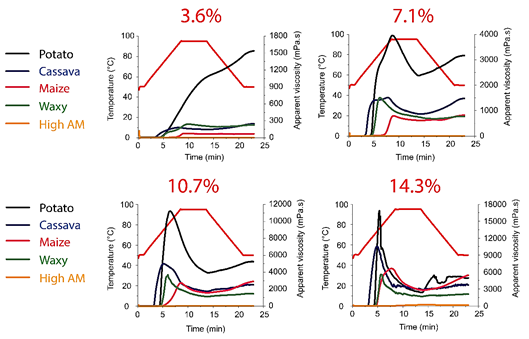
Figure 3 Pasting properties using the RVA standard temperature protocol (50-95-50 °C) and four starch concentrations (3.6%, 7.1%, 10.7% and 14.3%) of the different starch samples.
In general, the obtained results, for the same starch source, presented an increasing apparent viscosity (AV) with increasing starch concentration. However, not all the pasting behaviour and properties parameters showed a direct correlation with the starch concentration, being also dependent of the process conditions, the source, the interaction forces, among others, as discussed and described as follows.
At the standard concentration (10.7% of starch), the analysed samples presented an expected profile, typical for starches at those conditions (Figure 2and3), being the Peak AV per sample ordered as: potato > cassava > waxy maize > maize > high amylose maize. This behaviour was highly influenced by the starch intrinsic characteristics, as their granules size and morphology, as well as the amylose/ amylopectin ratio and their respective chain lengths (Jane et al., 1999; Tester & Morrison, 1990). Therefore, at this point, it is important to highlight some of those intrinsic characteristics of the starch here evaluated, which will be useful to understand the observed results. On the one hand, as described in Figure 1, the starch source according to their mean diameter (D[3,4]) was ordered from largest to smallest diameter as follows: potato > waxy maize > cassava > maize > high amylose maize. On the other hand, according to their apparent amylose content can be ordered as follows: high amylose maize (~72%) > maize (~28%) = potato (~28%) > cassava (~20%) > waxy maize (~2%).
As can be observed, no direct correlation can be done among the values of peak AV, the size or apparent amylose content values of the samples. In fact, the swelling behaviour of each starch granule is a complex relation of the amylose/amylopectin balance and their sizes, as well described and discussed in the literature (Jane & Chen, 1992; Jane et al., 1999; Tester & Morrison, 1990). An illustration of the relationship between the main intrinsic characteristic of the starches and their pasting properties can be found in Figure 7 (Principal Component and Classification Analyses discussion).
The Peak AV pattern (Figure 3andTable 1), however, was not maintained when the starch concentration varied. In fact, even among the samples with “predictable” behaviour, as the ones presenting increasing AV with increasing starch concentration, the increase was not linear or equivalent for the starch samples. By evaluating each starch source separately, we can observe some interesting patterns.
Potato starch, for example, presented a “non-standard” shape in its pasting curve at the lower starch concentration (3.6%), without the characteristic “Setback” and “Breakdown” regions (Figure 3). This behaviour occurred only with the potato starch, and thus it cannot be explained only by the lower interactions among the starch granules, which is typical at low concentrations (Biliaderis, 2009;Booth & Bason, 2007). This demonstrates that the interactions of the leached molecules during cooling were expressive, even considering the negligible granules’ swelling during pasting. It is interesting to mention that this concentration (3.6%) is closer to the typical used in the industry, such as for mayonnaise (Mun et al., 2009), stirred yogurt (Wong et al., 2020), salad dressings (Bortnowska et al., 2014; Franco et al., 1997) or processed meat products (Pietrasik & Soladoye, 2021) for example, although the standard protocol uses a concentration of 10.7%, which is, in general, much higher than the concentration of industrial application when starch is used as additive. On the other hand, when starches are used as main ingredients, for example in noodles (Li et al., 2020) or bakery products the starch concentration is much higher than 10.7%.
Table 1 Main parameters of the pasting properties of each starch source under different analysis concentration and using the RVA standard temperature protocol (50-95-50 °C). Average ± standard deviations. AV = apparent viscosity. Samples followed by the same letter do not differ significantly at 95% of confidence (statistical analysis performed for the same starch source)
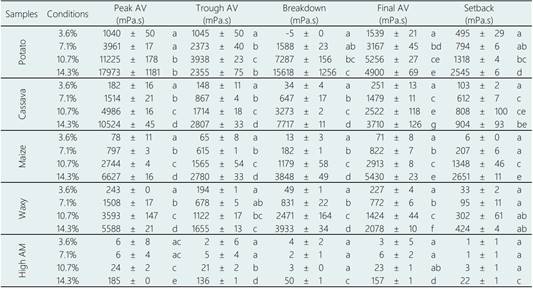
Therefore, we highlight that decisions of industrial application based on the starch characteristics obtained using standard concentration may not suit the real necessities, leading to misinterpretations.
Another aspect regarding the potato starch was its behaviour at the highest concentration (14.3%). At this condition, the sample presented lower Trough AV and Final AV if compared to the 7.1 and 10.7% samples (Table 1). These results can be related to a possible lower gelatinization degree of the most concentrated sample, due to the lack of available water and/or due to the potato starch bigger granules (explaining why this behaviour was not observed for the other starch sources). The less swelled/gelatinized starch granules probably influenced in a lower extend the AV of the system, if compared to the more gelatinized granules. In fact, this could be proved by optical microscopy (Figure 4) where at the highest concentration (14.3%), incompletely gelatinized granules were observed.
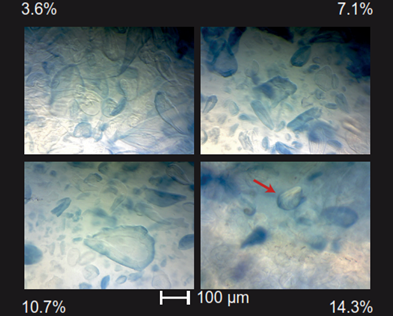
Figure 4 Light microscopy* images of the potato starch gel at different starch concentrations (3.6, 7.1, 10.7 and 14.3%) after the RVA analysis using the standard temperature protocol (50-95-50°C). The white line measures 100 µm. The red arrow indicates an incompletely gelatinized granule. *Light microscope (model L1000, Bioval, Curitiba, Brazil) with a 20 W halogen lamp. Lugol solution (1% I2 and 2% KI) was used to better visualize the gels. The magnification was of 400x and a portable camera of 1.3 megapixels was used to obtain the images.
Regarding the cassava starch, at 3.6 and 7.1% of starch concentration, its Peak AV was lower than its Final AV, condition that is inversed by increasing the starch concentration. To give a possible explanation to this behaviour, we first need to recall that the RVA analysis measures the apparent viscosity of the system during a heating-cooling process. During this process, the starch granules suffer irreversible changes, and not only their leached amylose and amylopectin molecules but also the remnant granules, their fragments and ghosts are interconnected and form a complex network (“gel”) that changes the system’s characteristics. Keeping that in mind, it is possible to say that the effect of cooling in the network formation of cassava starch is more significant at lower (3.6 and 7.1%) than at higher concentrations (10.7 and 14.3%).
Regarding the waxy and normal maize starches, the importance of the starch/water concentration becomes even more evident. For example, the waxy maize is usually mentioned as presenting a higher Peak AV if compared to the normal maize starch. However, this statement can only be considered true at the lower concentrations (3.6, 7.1 and 10.7%), since at 14.3% the normal maize presented a higher Peak AV value if compared to the waxy sample (Table 1). The amylopectin molecules are usually related to the peak AV observed in the RVA analysis (Liu, 2005), which explains the waxy maize starch higher peak AV if compared to the normal maize starch. However, the amylose/amylopectin ratio is a complex and important parameter that can influence more the starch gelatinization behaviour than the amylopectin content by itself (Jane & Chen, 1992). This ratio can possibly explain why at 14.3% the normal maize starch presented a higher apparent viscosity if compared to waxy samples. On the other hand, regarding the Final AV, an opposite behaviour was observed, and the waxy starch only showed a higher Final AV than the normal maize starch at 3.6%. This result also can be explained by the amylose/amylopectin ratio of the maize samples, but in this case, the amylose content played a more important role (since it is more related with the Final AV than the amylopectin molecules).
The high amylose maize starch presented a negligible AV variation after the RVA tests. Only at 14.3% its AV variation was minimally measurable (being comparable to the AV of the cassava and waxy maize samples at 3.6%, Figure 3), probably due to its small but present amylopectin concentration. This lower AV is due to the incomplete gelatinization of the high AM maize starch under the RVA pasting conditions (gelatinization temperature < 100 °C). As discussed in the literature (Tester & Morrison, 1990), the amylopectin molecules have a major contribution to the starch swelling, while the amylose inhibits it. In fact, the high amylose starches (> 50% of amylose content) are usually reported to require a high temperature (> 130 °C) to be completely gelatinized (Case et al., 1998; Fredriksson et al., 1998; Jane et al., 1999; Rincón-Londoño et al., 2016).
In summary, when comparing the AV values of starch, each source presented a different behaviour in relation to the starch proportion variation. Considering the peak AV, for example, the cassava starch showed a decrease of ~70% on the peak AV value when the starch concentration was reduced from 10.7% to 7.1%, and an increase of ~111% when the concentration was increased from 10.7% to 14.3%, while the other starch samples showed different behaviours. At the same conditions, the maize starch presented a decrease of ~71% and an increase of ~141%, the waxy maize starch a decrease of ~58% and an increase of ~54%, and the potato starch a decrease of ~65% and an increase of ~29%. Therefore, all those results for each starch source show that the starch properties are not easily predictive, and once again that 10.7% of starch concentration is not always representative for the starch behaviour in all possible applications.
Gel strength
Figure 5 illustrates the gel strength of the samples obtained after each RVA test. The high amylose maize starch presented a weak gel, due to its incomplete gelatinization, as previously discussed for the RVA analysis, therefore these results are not shown in Figure 5.
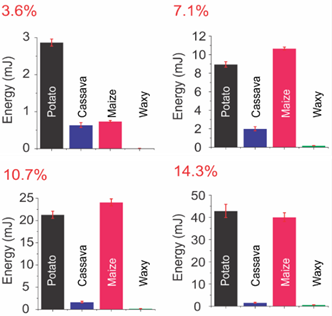
Figure 5 Gel strength of the starch gels obtained after the RVA tests using the standard temperature protocol (50-95-50 °C).
Furthermore, since no significative differences were observed when comparing the samples obtained through the different RVA temperature protocols (using 40 and 50 °C), only the results referent to the gels obtained using the RVA protocol at 50 °C are presented in Figure 5. However, all the gel strength results at both temperatures were considered for PCA and HCA (Figure 6and7) to better correlation among all variables in this study.
The gel strength for the waxy maize starch presented an extremely weak gel with a negligible strength (with its highest value of ~0.6 mJ). Considering that the gel formation depends on a complex network between amylose and amylopectin molecules that leached the granules during the gelatinization, as well as the remnant granules and their fragments, it is possible to say that the weak texture presented by the waxy starch was due to the lack of amylose molecules in this sample. In fact, the amylose molecules are highly correlated to the gel formation after cooling (Ai & Jane, 2015; Miles et al., 1985).
On the other hand, the cassava starch presented smooth and elastic gels, with an extremely low resistance to the penetration test (highest value of ~2 mJ in Figure 5). In this case, the low amylose content (~20%) can also be related to this weak gel. However, another factor can be involved, as the high amylopectin chain-length, which can impair to some extent the molecular re-association of the cassava sample after cooling.
The gel strength of the potato and the maize starches was similar in almost all starch concentrations (except at 3.6%), despite the high differences among their pasting properties. Both presented the highest gel strength values among all samples. This can be attributed to their similar amylose content (~28%), which is mainly related to the short-period gel formation (Ai & Jane, 2015; Miles et al., 1985). On the other hand, the difference observed in their pasting properties can be attributed to their different granule size and phosphorus content. The potato starch phosphorus content (~800 mg/kg, Castanha et al. (2017)) is much higher than the other starch sources and is frequently attributed to enhance the apparent viscosity (Lu et al., 2012).
In fact, the pasting profile of the starch samples cannot be used as the only parameter for prediction of the gel formation and/or strength after cooling. In the case of the gel, in special regarding its development after a short storage period, the amylose content and its leaching of the granules plays an important role (Ai & Jane, 2015; Leloup et al., 1991). The cassava starch, for example, presented the second highest peak AV of all samples, in all concentrations. However, its gel presented a negligible strength, illustrating that the pasting profile cannot be used to predict the behaviour of the starch gel after cooling and storage.
The exact role that each parameter plays and how much they influence the final characteristics of the starch samples is difficult to be determined. However, the correlation between the starch source, structure, properties, and processing conditions can be evaluated through statistical analysis, as follows.
Hierarchical cluster analysis (HCA) and Principal Component and Classification Analyses (PCA)
Figure 6 illustrates the Hierarchical Cluster Analysis (HCA) of the potato, cassava, waxy maize, and normal maize starches at different concentrations considering both 40 and 50 °C. The high amylose maize starch was not considered in the HCA analysis due to its outlying gelatinization behaviour.
Figure 6 illustrates the dendrogram with an added green line indicating the stopping point after the 28th stage of the clustering procedure, which was defined from the agglomeration schedule eliminating the last four stages (stages 29 to 32). Five clusters were revealed, whose classification criteria and interpretation were mainly related to the starch source, concentration and pasting properties. In general, the first clustering criteria (similarity) was the type of starch source with the same concentration, initially grouped in pairs despite the different temperature, with exception of potato starch 14.3%. This shows the little effect of the temperature and the higher effect of starch concentration described for most cases (Figure 2, section 3.1). However, only in potato starch 14.3%, the effect of temperature was significant, observing a cluster for each temperature (40 and 50 °C). The dissimilarity was mainly correlated with their different (p< 0.05) values of Final AV (2596 ± 586 and 4900 ± 69) and Setback (376 ± 488 and 2545 ± 6) parameters at 40 °C and 50 °C, respectively. The next cluster was composed for samples of starch from Cassava (14.3%) and Potato (10.7%), the grouping criteria of this samples could be explained by the similar values of peak, trough, breakdown, and final AV. Finally, the last cluster include the samples that showed the lower values of pasting properties parameters evidenced in Figure 2-3 and Table 1.
The Principal Component and Classification Analyses (PCA) results are shown in Figure 7, which is the projection of the variables on the factor-plane (1x2) and represented 70.2% (explained variance) of the whole information.
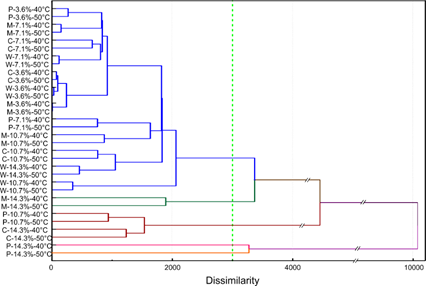
Figure 6 Hierarchical Cluster Analysis of the different starch sources classified by their main parameters of the pasting properties. The vertical discontinuous line indicates the suggested stopping location, which determined the formed clusters. Legend: P = potato, M = maize, C = cassava and W = waxy maize. The high amylose maize is not represented due to its outlying gelatinization behaviour.
The factor 1 (PC 1), with factor-variable correlations r ( |0.68|, was represented by the variables of pasting properties parameters (Table 1), the energy (or gel strength, Figure 5) and starch concentration. Factor 2 (PC 2) was mainly represented (r ( |0.66|) by the starch source, granule volume and area (data from the PSD analysis, Figure 1).
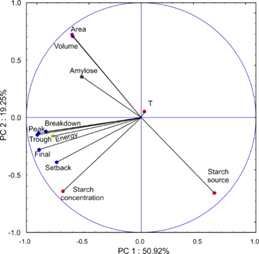
Figure 7 Principal component Analysis represented on two principal components (70.2% of explained variance).
The results showed that starch concentration is the variable that presented a higher impact on the pasting properties and gel strength. In addition, it was demonstrated that the particle volume and area were positively correlated (r > 0.7) with the starch source. It is in accordance with the observed in Figure 1 about the different starch granules’ morphology and PSD in function their source. Regarding the amylose content and temperature, they were not strongly represented in the factor plane (1x2). Therefore, their influence was not evidenced.
Summarizing, considering all the results and the literature data, the starch gelatinization is a complex phenomenon that is affected by several intrinsic and extrinsic parameters. Therefore, despite the need of a standard protocol to allow a fair comparison among different starch samples, native or modified, the results obtained through these typical analyses cannot be settled as unique or always true. In fact, the starches behaviour under different processing conditions are difficult to predict and control, and worth be individually investigated.
4. Conclusions
In this work, different starch concentrations showed influence on the pasting properties and gel strength. However, the effect of using a different initial and final RVA testing temperature does not present a significant effect - in the exception of highest starch concentration (14.3%). Further studies considering a higher temperature variation must be performed in order to better evaluate the effect of this parameter on the starch samples. It was demonstrated that using only at RVA standard concentration of 10.7% may not be appropriate to evaluate the starch behaviour in all possible applications. By varying the starch concentration, not only the apparent viscosity value during RVA varied, but also the behaviour and shape of their pasting curves. Furthermore, the different starch concentrations influenced the strength of the obtained gels.
The results provide new useful data for the characterization of different starch samples under non-standard conditions of analysis, expanding the results that are available for future reference of the analysed starches. Since the starch has wide ranges of application (conditions, products), it is recommended to continue researching their properties and behaviour at the actual application conditions to facilitate the choice and/or predict the results.