1. Introduction
In agro-industrial processes, large quantities of by-products are generated that are not of interest and as they have not economic value, only a part is reused (Struck & Rohm, 2020). When they are discarded, during their decomposition they produce gases that cause impact to the environment, in addition to being a source of microorganisms that are harmful to the health of people and other living beings (Shirahigue & Ceccato-Antonini, 2020; Sumaya-Martínez et al., 2019). The by-products that are also produced from the consumption of raw materials, are an important source of antioxidants, represented especially by phenolic compounds (Velderrain-Rodríguez et al., 2019), which, based on Siacor et al. (2020), their demand is increasingly in the international market, projecting their annual economic value to increase by 7.2% from 2019 to 2025. Mango (Mangifera indica) is one of the most widely produced and consumed fruits (Mwaurah et al., 2020), due to its delicious flavor, aroma, color and nutritional value (Lebaka et al., 2021) and according to FAO, its production in 2018 was 52.08 million metric tons (Marcillo-Parra et al., 2021), representing more than 50%, in terms of tropical fruits (Alañon et al., 2021). It has great antioxidant potential as it contains compounds such as retinol (El-Desoukey et al., 2020), ascorbic acid, carotenoids (Ribeiro et al., 2008) and phenolic compounds such as mangiferin (Figure 1), anthocyanins, kaempferol, quercetin, catechin, rhamnetin, gallic acid, benzoic acid, ellagic acid, tannins, flavonols, benzophenone and their derivatives (Dorta et al., 2014; Mwaurah et al., 2020), which are mainly found in the peel and the seed (Ribeiro et al., 2008).
Despite its importance, during its processing and/or consumption, especially in juice, concentrated pulp, jam, puree, nectar or other related product (Rojas et al., 2018), high volumes of residues such as seeds and peels are generated, ranging from 35% to 60% of the weight of the fruit, although they contain bioactive compounds of interest (Meneses et al., 2015; Sumaya-Martínez et al., 2019), especially from the phenolic group as shown in Figure 2.
Considering the previous, besides containing the mentioned vitamins and also, tocopherol (Sumaya-Martínez et al., 2019), synergistically, they provide the by-products with excellent pharmacological attributes such as: antiatherogenic, antitumoral (Ribeiro et al., 2008), anti-inflammatory, antimicrobial and anticancer being represented especially by mangiferin, the main compound present in the mango (Castro-Vargas et al., 2019; Meneses et al., 2015). Due to their properties, they present a great interest in the food industry, being able to use them as natural additives in replacement of synthetic ones, obtaining healthier foods and preventing malnutrition (Mwaura et al., 2020). In this context, by giving them an added value, in addition to providing an improvement in economic terms (Ordoñez-Torres et al., 2020; Shirahigue & Ceccato-Antonini, 2020), their correct use would help reduce environmental and health problems caused by their decomposition (Sumaya-Martínez et al., 2019).
As shown, mango peel and seed have a large number of phenolic families and in addition to those detailed, they contain derivatives of the same. For example, Ordoñez-Torres et al. (2020) identified in the peel, compounds such as galloyl glucose, gallic acid 3-O-gallate, tetra-galloyl glucose, among other derivatives. Likewise, Lebaka et al. (2021) indicate that the seed presents polyphenols such as cyanidin, mangiferin gallate, homomangiferin, isomangiferin, isomangiferin gallate, and rhamnetin 3-0 galactoside/glucoside. Despite the characteristics and phenolic properties of mango by-products, Lim et al. (2019) indicate that they can be easily degraded during processing and storage and can suffer darkening and even alterations in their antioxidant qualities. Therefore, El-Messery et al. (2021) mention that conservation technologies such as microencapsulation should be applied.
In addition to preserving the phenolic characteristics of external factors such as oxygen, water, light (Velderrain-Rodríguez et al., 2019), pH and moisture, it also has benefits such as masking odors, enhancing flavors, and facilitating their use in desired conditions (Siacor et al., 2020).
Bioactive compounds are becoming increasingly popular in the industry, since they have diverse qualities and benefits for humans, and even more so if they are derived from agroindustrial waste, since adding value to them would contribute economically and mitigate the pollution they usually generate. In this regard, the objective of this review was to gather information on the antioxidant and antimicrobial characteristics of phenolic compounds from mango peel and seed, as well as to report on their potential pharmacological applications and especially as an additive in the food industry.
2. Mechanism of antioxidant and antimicrobial action
2.1 Antioxidant potential
According to several studies, free radicals oxidize DNA, proteins, lipids and other compounds in living organisms, specifically in humans, being related to the appearance or progression of cancerous, cardiovascular, respiratory and even neuronal diseases (Huang et al., 2018). These effects can be inhibited by flavonoids that as mentioned by Ochoa & Ayala (2004), they have 20 and 50 times more antioxidant activity than ascorbic acid and tocopherol, respectively.
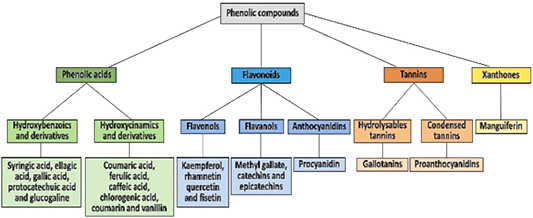
Figure 2 Classification of phenolic compounds contained in mango peel and seed. Based on information from Bai et al. (2018); Ben-Othman et al. (2020); Dorta et al. (2014); Masibo and He (2008); Mercado (2014).
Mango has attracted the attention of researchers for its quantity and variety of polyphenols, and its antioxidant potential. According to study of Mercado (2014), it was concluded that mango pulp has higher total phenolic content than fruits such as grape, blackberries, strawberries and pineapples, and therefore a higher antioxidant capacity: 174.3 mg of vitamin C equivalent/100g for DPPH (2,2 diphenyl-1-picryhydrazyl) assay. Considering by-products, Ribeiro et al. (2008) focused on the mango variety 'Ubá' and in their research, the peel presented a total phenolic content of 57.24 mg/kg of dry matter and 82.54 mg/kg in the seed. Likewise, the peel and seed extracts showed 89 and 60.7% radical inhibition by the DPPH method, in addition to a reducing power of 0.97 and 1.05, respectively, at a concentration of 5000 ppm.
In the investigation of Soong & Barlow (2004), the mango seed had higher antioxidant activity than tamarind and avocado. The values using the Folin-Ciocalteu reagent (FCR) were 117, 94.5 and 88.2 mg of gallic acid equivalent (GAE)/g for mango, avocado and tamarind, respectively.
Previous studies compile by Mercado (2014) reported higher total phenol content in mango seed extracts (2572 mg GAE/g) compared to 1144 mg GAE/100g in apple, 2335 mg GAE/100g in grapefruit and 820 mg GAE/100g in kiwi. Furthermore, the antioxidant capacity of mango seed (4188 μmol Trolox equivalent (TE)/g) and peel (1846 μmol TE/g) is very high compared to 462 μmol TE/g from avocado seed, 75.4 μmol TE/g from passion fruit peel and 58.4 μmol TE/g from papaya peel. Particularly, in the research of Meneses et al. (2015), they determined the phenolic content of the extract of a mixture of Tommy Atkins and Haden mango peels, with the addition of pulp, which was 29545 mg GAE/kg.
Vega-Vega et al. (2013) indicates that phenolic compounds protect the human cells against oxidation factors. Masibo & He (2008) mention that they have prevent the oxidative damage of the DNA, to eliminate the free radicals, preventing alterations in the organism and specifically, degenerative diseases and aging. According to Minatel et al. (2017), phenolic compounds stabilize free radicals by giving up hydrogen atoms or electrons, as well as chelating metals important for oxidation enzymes. Another mode of antioxidant action of phenolic compounds is their inhibitory effect on the formation of hydroperoxides (Maqsood et al., 2014) from the lipid peroxidation of, for example, a monounsaturated fatty acid, preventing its oxidation by free oxygen radicals (commonly catalyzed by metal ions) or by enzymes such as lipoxygenases (Figure 3).
Mangiferin, the major phenolic component present in mango residues, is the main cell protector and according to Masibo & He (2008), its activity as an antioxidant agent is present even in low concentration.
2.2. Antimicrobial potential
Pathogenic microorganisms in humans and animals are increasingly resistant to drugs due to their misuse or overuse. These are especially synthetic which also cause side effects in the consumer's body, therefore, there is a need for new high spectrum antimicrobial agents with few or no side effects (Huang et al., 2018), which could also be used in food, since microorganisms are the main cause of the deterioration of their characteristics, altering their quality.
Phenolic compounds have a high antimicrobial effect by causing structural or functional damage in the cell membrane, due to the adsorption of phenols, the interaction with enzymes, with hydrophilic amino acids of proteins, causing an alteration of the pH and electrical potential (Raybaudi-Massilia et al., 2009), the deprivation of metallic ions, this by the action of phenolic acids, specifically. Also, the coagulation and leakage of the cellular content by the effect of gallic acid (Mercado, 2014), the formation of harmful complexes by the synergistic action with flavonoids (Bouarab-Chibane et al., 2019), alteration of the genetic system and therefore, death (Figure 4). According to several studies, the extract of the mango seed Haden, Ataulfo and Tommy Atkins had an antimicrobial effect against Salmonella spp., Escherichia coli O157:H7, Listeria monocytogenes, Staphylococcus spp., among others. Mango var. Haden specifically demonstrated impact against bacteria such as Bacillus cereus, B. subtilis, Pseudomonas aeruginosa, Vibrio vulnificus, Salmonella typhi and Clostridium spp. (Vega-Vega et al., 2013). Similarly, mango peel extract showed a high effect against Staphylococcus aureus, E. coli, P. aeruginosa, B. cereus, Candida albicans (El-Desoukey et al., 2020), S. typhi, Shigella spp., Enterobacter spp., Aspergillus niger (Thambi et al., 2016), Colletotrichum gloeosporioides, Sclerotinia sclerotiorum, Fusarium oxysporum and Mucor sp (Rojas et al., 2018).
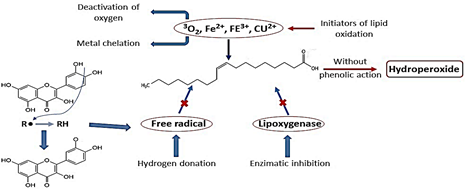
Figure 3 Effect of phenolic compounds preventing the formation of hydroperoxide from oleic acid by various mechanisms. Adapted from Maqsood et al. (2014).
Dorta et al. (2016) conducted tests on the fungal activity of the seed and peel of Keitt, Sensation and Gomera mangoes. Their extracts showed inhibitory effect against multiple yeasts of the genera Saccharomycodes, Lodderomyces, Hanseniaspora, Candida, Dekkera, Metschnikowia, Schizosaccharomyces, Zygosaccha-romyces and Pichia. According to the analyses performed, it was determined that this property was related to the presence of gallates, gallotannins and proanthocyanidins.
In an in vivo experiment, Gómez-Maldonado et al. (2020) using a solution with of Ataulfo mango seed extract (3 g/L) with 461.7 mg GAE/g and DPPH activity of 2919.0 μmol TE/g, achieved, by immersion of mango fruits, total mycelial inhibition of Colletotrichum brevisporum (inoculated at 105 spores/mL) at 9 days and of its spores, at 20 h.
According to the background, the antibacterial effect of phenolic compounds in mango by-products is more efficient against Gram-positive ones, confirmed by Huang et al. (2018) which argue the low susceptibility of Gram-negative due to the lipopolysaccharides present in their membrane, making them more resistant, repelling or slowing down the interaction with polyphenols.
3. Application of phenolic compounds
The phenolic potential of mango by-products, (19.06 and 23.9 mg GAE/g, and 93.89 and 95.08% inhibition of the DPPH radical, in the peel and seed, respectively, according to Ashoush & Gadallah, 2011), qualify them as key ingredients in the production of pharmacological products since, according to several studies, they have also demonstrated other properties such as antimutagenic activity (Kantrong & Eshtiaghi, 2018), anti-inflammatory, antiallergic, antidiabetic, antihyperlipemic antiproliferative, antiplatelet, antiaging, as an immunomodulator and even, against neurological diseases, respiratory, hemorrhagic (Lebaka et al., 2021) and defense against breast, liver, leukemia, cervical, prostate, lung and colon cancer (Alañon et al., 2021). In addition to those mentioned, by presenting functional characteristics such as antioxidant, biopreservative, coloring and flavoring, they are qualified as ideal ingredients for the food industry (Mwaurah et al., 2020), specifically in the field of nutritional products (Marcillo-Parra et al., 2020), also promoting a culture of maximum utilization of food (Zepeda-Ruiz et al., 2020).
3.1. Disease prevention
3.1.1. Anti-cancer and cardiovascular disease
Mercado (2014) concluded in her research that gallo-tannins from mango seed extract had antiproliferative effect against cancer cells of liver (HepG2), breast (MDA-MB-231) and leukemia (HL-60).
Studies indicate that proanthocyanidins and tannins have the potential to prevent digestive and other internal organ cancers. They also prevent oxidation of low-density lipids (LDL) and blood clotting, thus avoiding cardiovascular diseases (Vermerris & Nicholson, 2006).
Similarly, research has shown that there is a high correla tion between polyphenol levels, especially flavonoids, and the prevention of cancer and cardiovascular diseases, platelet disorders, etc., as well as boosting the immune and metabolic systems (Minatel et al., 2017).
According to Gordo (2017), flavonoids prevent cancer by blocking the cycle of cancer cells at a certain stage and apoptosis, in addition to preventing oxidation, inducing detoxification enzymes (Phase I and Phase II).
Martínez-Flórez et al. (2002) conducted an in vitro experiment in which they concluded that quercetin prevents breast cancer, possibly due to its powerful capacity to inhibit aromatase, which is responsible for the biosynthesis of estrogens. It also increases the concentration of glutathione in the body, which has an impact on the prevention of lipid peroxidation caused by iron.
According to Quiñones et al. (2012), polyphenols have ef fectively inhibited the angiotensin converting enzyme (ACE), a potent vasoactive and blood pressure regulator, which would justify the vasodilatory and cardioprotective effects of phenols. In addition, they have an antithrom botic effect since anthocyanins inhibit platelet function, preventing the formation of clots inside the arteries and veins.
3.1.2. Anti-inflammatory
Hole et al. (2012) highlight the anti-inflammatory activity of caffeic, coumaric, synapic and ferulic acid in a synergistic way on the activity of the protein complex (NF-κB), a complex involved in inflammation, stress responses, differentiation and activation of immune cells. Similarly, Tanase et al. (2019) stated that this property of phenols is due to their mechanism of blocking the overproduction of tumor necrosis factor (TNF-α), a protein that also executes functions related to inflammation.
Flavonoids avoid the risk of inflammation (Vermerris & Nicholson, 2006) and together with proanthocyanidins help in the absorption, protection and prolongation of the functions of vitamin C, such as the preservation of collagen (a protein that forms tissues) against enzymatic activity, damage by free radicals and the synthesis of inflammatory agents (Ochoa & Ayala, 2004). Quercetin has the same potential due to its cyclooxygenase (COX) and lipid peroxidation (LPO) inhibitory effect (Quiñones et al., 2012).
3.1.3. Neuroprotector
The reports of Alañon et al. (2017) highlighted the effect of phenolic compounds against neurodegenerative problems. Lemus-Molina et al. (2009) corroborated that information when they determined in vivo in the cerebral cortex of rats that mangiferin successfully protected neuroblastoma cells from oxidation deliberately induced by hydrogen peroxide. Similarly, according to Masibo & He (2008), mangiferin reduced the intracellular concentration of Ca2+ in the brains of gerbils, demonstrating its property against neurological diseases, especially preventing neuronal death.
The effect of quercetin, caffeic acid, catechin and epicatechin on the inhibition of 5-S cysteinyldopamine (Alañon et al., 2017), a neurotoxin that influences the progression of Parkinson's, has been reported.
Regarding flavonoids, Alañon et al. (2017) mention that they have neuroprotective action, due to their influence in modulating cell signaling, to suppress neuroinflammation by reducing the release of cytokines, decreasing proinflammatory factors and transcription pathways.
3.1.4. Antidiabetic
Bhuyan & Basu (2017) described the anti-diabetic mechanism of phenolic compounds. They inactivate α-amylase, α-glucosidase, maltase-glucoamylase and sucrase-isomaltase, fundamental enzymes in carbohydrate degradation. Martín et al. (2018) added that chlorogenic acid, caffeic, ferulic, and quercetin affect the transport of carbohydrates and also influence as regulators in the release of satiety hormones. Furthermore, its effect was found to be even greater than that of conventional synthetic drugs and without the risk of causing any negative effect on the consumer. Based on in vivo animal studies, non-flavonoids help re duce the production of inflammatory agents such as monocyte chemoattractant protein-1 (MCP-1), cyclooxygenase-2 (COX-2), interleukin-1 (IL-1β), interleukin-8 (IL-8) or nitric oxide synthase (iNOS), important factors in the development of diabetes (Bhuyan & Basu, 2017). According to the information collected by Ballard & Junior (2019), flavonoids have action in increasing insulin secretion, metabolic regulation of glucose and flavanones especially, improve insulin resistance.
3.1.5. Other health benefits
In vitro studies report that flavonoids and anthocyanins reduce the viability of adipocytes, preventing the accumulation of triglycerides, improving the oxidation of fatty acids and reducing inflammation, demonstrating their effect against obesity (Ballard & Junior, 2019).
Phenolic compounds also have an effect on the stimulation of the immune system. Research have determined that cinnamic and caffeic acid activate macrophages. Epicatechin gallate enhances the T cell; ferulic, chlorogenic and coumaric acids increase the secretion of cytokine interferon- γ (IFN-γ) (Bhuyan and Basu, 2017), both of which are important in the regulation of the immune system. Similarly, it was shown that phenols can act in the inhibition of endothelial dysfunction and mitochondrial dysfunction (Soto-Vaca et al., 2012).
Based on a study cited in Masibo & He (2008), mangiferin reduces bad cholesterol and triglycerides in plasma and provides protection against radiation-induced disease. In another similar study, according to Zepeda-Ruiz et al. (2020), the consumption of the different parts of the Ataulfo mango for two months helped to improve the lipid profile and the antioxidant activity of plasma in people without any disease.
Also, El-Messery et al. (2021) demonstrated in vivo, using rats, that by giving them a milk drink with microcapsules of phenolic extract of mango peel, it was possible to increase the level of antioxidants and catalase, in addition to reducing the activity of lipid peroxidation, alalanine transaminase, aspartate transaminase and alkaline phosphatase decreased, thus preventing oxidative stress disorders.
3.2. Use in the food industry
3.2.1. Modification or enhancement of sensory qualities
Bitterness and astringency in foods, an important characteristic in certain products, such as wine, beer (de Oliveira et al. 2014), nuts, chocolate, coffee, tea, which are conferred by anthocyanidins or condensed tannins (Soto-Vaca et al., 2012), or volatile polyphenols such as vanillin (El Gharras, 2009), could be enhanced and improved to positively influence consumer choice.
One of the factors influencing the taste of aged alcoholic beverages is the changes in phenolic compounds, for example, Soto-Vaca et al. (2012) mention that during the aging process of whiskey in barrels, ellagitannins are degraded and tannins are further oxidized, generating mixtures of castalagins, an ellagitannin that contribute to the flavor of the drink. With respect to tannins and their derivatives, Lebaka et al. (2021) indicate that 75% is in hydrolyzable form, so that prior to its use in food, it is necessary to process them, also reducing a possible risk of toxicity.
Anthocyanins, flavanols and flavones are important pigments that also have an effect on food flavor. Proanthocyanidins can be used as copigments to produce red colors together with anthocyanins (de Oliveira et al., 2014). Anthocyanins are very reactive, as during the food processing process, they can affect the color, forming a wide variety of shades (El Gharras, 2009).
3.2.2. Other applications as additives
Phenolic compounds could be used as biopreservatives, enriching the antioxidant and antimicrobial capacity of fresh produce such as fruits and vegetables and of functional foods in general, free of synthetic additives which is currently of concern to the population because of their influence on health. For example, they have been effectively employed in the prevention of lipid oxidation of meat products such as hamburgers, sausages, nuggets and even margarine (Martillanes et al., 2017). Specifically, catechins are used as an additive to prevent oxidation of oils and fats, to reduce the microbial load and as a fortifier/enrichment of various food products (El Gharras, 2009). Concerning sunflower oil, in the research of Sánchez-Camargo et al. (2019), employing Sugar mango peel extract, at a ratio of 200 to 1000 ppm, which contained 64.8 mg GAE/g and carotenoids (1.9 mg all-trans-β-carotene/g), it was possible to prevent its oxidation in an efficient and natural way.
Martillanes et al. (2017) mention that polyphenols could be incorporated in films and edible coatings, even in food packaging, obtaining an active packaging that prolongs its useful life and according to Arroyo et al. (2019), they could also enhance the physicochemical, nutritional and sensory characteristics with certain modifications or mixtures with other components that are considered.
Pacheco-Ordaz et al. (2017) concluded in their research that catechin, gallic, vanillic, ferulic and protocatechuic acid from mango by-products promoted the development of Lactobacillus rhamnosus and L. acidophilus, in addition to inhibiting the spread of E. coli and Salmonella typhimurium. Phenolic compounds demonstrated their use as a probiotic enhancer which can be applied in yogurt, cheese, olives, sauerkraut and other functional foods. According to the research of Mwaurah et al. (2020), they propose that the flour of the mango seed can partially substitute the wheat flour due to its antioxidant activity (73% against 22.4% of the wheat flour), besides containing 44.21 UI/100 g of provitamin A, 1.03 and 0.45 mg/100 g of tocopherol and ascorbic acid, respectively, and other components which would help to enhance the nutritional, physicochemical and sensory characteristics of the bread or other food products.
A particular case is that of Pérez-Chabela et al. (2021), who added mango peel flour in different proportions in a yogurt, managing to inhibit undesirable microorganisms and helping the proliferation of lactic acid bacteria, in addition to containing high levels of dietary fiber (35.41%) and carbohydrates (40.08%), providing a good prebiotic and fermentative effect in the preparation of this type of product.
It should be noted that for the integration of mango by-products in different formulations or food matrices, as specifically shown in Table 1, it is recommended that the extract be raw to ensure greater bioavailability of the compounds, considering that, due to its susceptibility, further processing would generate high levels of degradation.
4. Methods of extraction and microencapsulation of phenolic compounds
4.1. Extraction techniques
There are many techniques for extracting phenolic compounds from mango peel and seed (Table 2). These must be easy to use, efficient, economical, and must extract the maximum number of compounds from the raw material, avoiding its degradation by the processing conditions (Mwaura et al., 2020).
Table 1 Application of phenolic compounds from mango by-products in food products
By-product/mango variety | Food product | Percentage of application | Main findings | References |
Peel/Zebda | Cookies | 10% | Increase in diameter by 2.89 mm, thickness by 1.51 mm, water absorption by 7.2%, dough development time by 8.5 min and stability by 18 min. Reduction in mixing tolerance by 50 BU and softening by 10 BU. Increase of 3.08 mg GAE/g and 53.76% DPPH activity. Overall acceptability improved, but not significantly. | Ashoush & Gadallah (2011) |
Peel/V.N.S. | Milk drink | 3%* | Doubling and tripling of bioavailability during in vitro digestion with values of 2.42, 6.36 and 8.25 mg catechin/g; 6.62, 46.53 and 64.61 mg rutin/g; DPPH activity of 1.1, 1.34 and 1.67 µmol TE/g; and FRAP of 0.85, 3.52 and 3.87 µmol TE/g, in the oral, gastric and intestinal stage, respectively, achieving a supplemented beverage. | El-Messery et al. (2021) |
Peel/Manila | Yogurt | 2% | Syneresis reduction of 0.7%, viscosity increase of 35.5%, acidity of 0.39 grams lactic acid/L and pH by 0.3. Sensory characteristics were affected, but not significantly, which did not influence the overall high consumer acceptability. | Pérez-Chabela et al. (2021) |
Seed/Nam-Dok-Mai | White shrimp | 0.5% | 90% decrease in polyphenol oxidase activity, total basic nitrogen (from 79.64 to 57.43 mg N/100 g), thiobarbituric acid reactive substances (from 1.38 to 0.98 mg malanoaldehyde/kg), psychrophilic bacteria index (from 6.2 to 5.65 log CFU/g), inhibited melanosis and prolonged shelf life during storage on ice for 12 days. | Sai-Ut et al. (2020) |
Peel/V.N.S. | 25 desserts** | N.S. | Optimum sensory performance in terms of appearance (4.2 to 4.9), color (3.9 to 4.9), texture (3.8 to 4.9), odor (4.2 to 4.8) and flavor (3.7 to 4.9), in addition to having an overall average acceptability of 4 to 5, taking into account that the hedonic scale was from 1 to 5, from "poor" to "excellent". | Thambi et al. (2016) |
Peel/Tommy Atkins | Kefir during fermentation | 5% | Increased antioxidant activity DPPH (113%) for 10 h, FRAP (153%) for 8 h, ABTS (21%) for 6 h and FRAP (43%) for 8 h, in addition to tripling lactic acid bacteria (up to 108 CFU/mL) during 12 h, helping to obtain a better probiotic product. | Vicenssuto et al. (2020) |
Peel/Ataulfo | Corn chips | 15% | Increase of 8 mg GAE/g and integration of 1950, 63 and 49 µg GAE/g of mangiferin, quercetin and rutin. Regarding digestive bioavailability, phenolic content and DPPH activity had an increase of 378 and 401% in the oral stage, 239 and 194% in the gastric stage, 320 and 182% in the intestinal stage. Likewise, there was no difference with respect to overall acceptability | Zepeda-Ruiz et al. (2020) |
V.N.S.: variety not specified; N.S.: not specified; FRAP: ferric reducing power; ABTS: (2’2-Azino-bis (3-ethylbenzthiazoline-6-sulfonic acid)); BU: Brabender unit, in pharynography; CFU: Colony Forming Unit
*In the form of microcapsules; **Desserts: Alooparatha, dosai, idiyappam, kanchipuram idli, mango chutney, mini idli, ragi adai, vegetable pasta, bottle gourd porial, cauliflower peas masala, channa masala, coconut rice, mango rice, paneer buter masala, sambar rice, tamarind rice, beet root halwa, custard, green gram payasam, keasri, sprouts salad, sundal, sweet pongal, vegetable roll and yogurt.
The solvent is essential; the most suitable according to Ribeiro et al. (2020) are ethanol, methanol, acetone, diethyl ether, ethyl acetate, isopropanol and their mixtures with water, increase the efficiency of the process especially because they have a polarity close to that of phenolic compounds, which facilitates the diffusion stage. Likewise, it is essential to use green solvents that, in addition to helping to obtain quality products, avoid environmental impact. Based on Pal & Jadeja (2020), there are deep eutectic solvents, which are based on natural products such as carbohydrates and organic acids, are low cost, non-toxic, non-flammable, recyclable and provide a significant quantity and quality of compounds during extraction. Some examples of mixtures used by the same authors in their study during the extraction of polyphenols from mango peel were choline chloride-sucrose, choline chloride-glycerol, choline chloride-lactic acid, choline chloride-malic acid and sodium acetate-lactic acid.
Briefly, the most commonly used extraction techniques are, in the first place, conventional extraction, also called solid-liquid or maceration extraction, where, quite simply, the solvent diffuses through the food using organic solvents. Despite being the most used due to its simplicity, it requires more time and quantity of solvent, which implies higher cost, in addition to the fact that this type of classical extractions, such as Soxhlet, by the use of high temperatures, thermolabile compounds are degraded (Meneses et al., 2015) and even more so polyphenols, which are very susceptible. To solve these drawbacks, other methods have emerged, such as ultrasound-assisted extraction, which uses frequencies (20 to 100 KHz) and vibrations that generate the degradation of the cell wall of the food, contributing to rapid extraction of phenols and avoiding their degradation by not requiring high temperatures, except for the conventional method (Tanase et al., 2019).
Another equally efficient technique is microwave-assisted, in which minimal amounts of solvent and time are used, focusing especially on the electromagnetic field (0.3 to 300 GHz), achieving that the kinetic energy produced by the waves, diffuses through the food matrix and achieving a high extraction rate (Pal & Jadeja, 2020).
Table 2 Studies on the extraction of phenolic compounds from mango peel and seed
By-product/mango variety | Type of extraction/solvent | Optimal process parameters | Main findings | References |
Seed/Manila | Ultrasound/ ethanol-water (80:20) | t: 30 min; T: 20 ºC; f: 20 KHz; P: 750 W; A: 50%; solvent/sample ratio: 20 mL/g | 461.7 mg GAE/g and 2919 μmol TE/g | Gómez-Maldonado et al. (2020) |
Peel/Keaw | Subcritical water/water | t: 40 min; T: 220 ºC; solvent/sample ratio: 80 mL/g | 99.69 mg GAE/g | Kantrong & Eshtiaghi (2018) |
Peel/Tommy Atkins, Haden and Kent | Maceration/ acetone-water (70:30) | t: 30 min; T: environmental; solvent/sample ratio: 50 mL/g | 2931, 6624 and 5149 mg GAE/100 g; 502, 682 and 600 mg CE/100 g; DPPH activity of 23, 53.9 and 49.9 mM TE/100 g, for the three varieties. | Marcillo-Parra et al. (2020) |
Peel/V.N.S. | Maceration and Ultrasonication/ ethanol-acetone (60:40) | t: 15 min, T: 20 and 25 ºC; solvent/sample ratio: 20 mL/g; f: 24 KHz (for ultrasound) | 205.08 and 1493.01 mg GAE/100 g, for both processes, respectively. Antioxidant activity in ultrasound alone was 3.18 and 7.18 mg TE/100 g for DPPH and ABTS. | Martínez-Ramos et al. (2020) |
Peel/Tommy Atkins and Haden* | Supercritical anti-solvent/CO2 | T: 40 ºC; Φ: 1 mL/min p: MPa | Recovery of 90%, obtaining 16.83 mg/g, identifying mangiferin, iso mangiferin, quercetin, kaempferol, among others, in addition to a DPPH test of 851.9 μmol TE/g. | Meneses et al. (2015) |
Peel/Ataulfo | Ultrasound-microwave/ methanol (50%) | t: 10 min; T: environmental; solvent/sample ratio: 5 mL/g; f: 2450 MHz for microwave and 25 KHz for ultrasound | 54.15 mg polyphenols/g and ABTS, DPPH and lipid oxidation inhibition activity of 98.52, 94 and 91.74%, respectively. | Ordoñez-Torres et al. (2020) |
Peel/Kesar | Microwave/sodium acetate-lactic acid-water (1:3:4) | t: 19.66 min; T: environmental; solvent/sample ratio: 59.82 mL/g; P: 436.45 W | 56.17 mg GAE/g, FRAP activity of 683 µmol ascorbic acid eq./g and 82.64% DPPH inhibition. | Pal & Jadeja (2020) |
Peel/Ataulfo | Maceration/water | t: 10 min; T: 121 ºC; solvent/sample ratio: 40 mL/g | 72.61 mg polyphenols/g. 2.18, 37.73 and 39.23 ppm to inhibit DPPH, ABTS and lipid oxidation by 50%. | Rojas et al. (2018) |
Peel/Sugar | Microwave/ ethanol (60%) | t: 90 s; T: environmental; solvent/sample ratio: 50 mL/g; P: 800 W | 52.08 mg GAE/g, 2.75 mmol ET/g, and of 6.47 μg/mL with DPPH assay. Mango skin extract provided high antiproliferative effect against HT-29 colon cancer cell line, after 24 h (CI 50 = 22.98 μg/mL). | Sánchez-Camargo et al. (2021) |
Mixture between pulp, peel and seed/Palmer | Homogenizer assisted/ethanol (70%) | t: 5 min; T: environmental; solvent/sample ratio: 29 mL/g; f: 20500 rpm | 354.4 and 258.7 mg mangiferin and hyperoside/kg | Zuin et al. (2020) |
V.N.S.: variety not specified; t: time; T: temperature; f: frequency; p: pressure; Φ: fluid injection flow; P: power; A: amplitude; CE: catechin equivalent; IC50: concentration of the extract, required to eliminate half of the DPPH radicals. *Mixed peels of both mango varieties, with pulp residues.
Emphasizing environmentally friendly techniques, extraction with supercritical fluids is the most appropriate, in addition to ensuring product quality. Mainly CO2 is used as it is safe, compatible with phenolic compounds and easy to eliminate afterwards (Sánchez-Camargo et al., 2019); additionally, achieving its supercritical phase is relatively simple as it requires low levels of temperature (31 ºC) and pressure (74 bar). There is also a method employing subcritical water (100 to 320 ºC, 20 to 150 bar), which is also sustainable, safe, efficient (Sánchez-Camargo et al., 2021) and especially, has a high penetration into the cell wall, considerably raising the recovery level of the compounds (Kantrong & Eshtiaghi, 2018). The previously mentioned techniques are the most employed in the extraction polyphenols and, specifically, of mango peel and seed, as shown in Table 1, but there are also others that involve improvements or mixtures of the common ones such as accelerated solvent extraction (Tanase et al., 2019), conventional homogenizer-assisted (Zuin et al., 2020), ultrasound-microwave assisted (Ordoñez-Torres et al., 2020), supercritical antisolvent (Meneses et al., 2015) such as pulsed electric field extraction (Pattnaik et al., 2021), high hydrostatic pressure (Sánchez-Camargo et al., 2021), pressurized fluid (Martínez-Ramos et al., 2020) and enzymatic (Mwaurah et al., 2020).
It should be noted that, besides the type of extraction and solvent used (including its concentration), there are many more factors that influence the outcome of the process (Shirahigue & Ceccato-Antonini, 2020), such as the nature of the feed, temperature, time, solvent/sample ratio, particle size, pH (Martínez-Ramos et al., 2020), agitation speed (Tanase et al., 2019) and specifically, depending on the process, other variables such as amplitude, power and frequency (Pattnaik et al., 2021).
What is remarkable in the extraction of polyphenols, besides their quality and quantity (56.23 and 28.33 g total phenols/100g of dry matter in the peel and seed of mango var. Keitt, respectively, according to the results of Dorta et al., 2014), is their subsequent use as an additive, taking advantage of their properties without affecting the quality of the food. For example, Mercado (2014) extracted the phenolic compounds from the Haden mango seed which showed a DPPH and ABTS antioxidant capacity of 2034.1 and 4205.7 μmol TE/g. The extracts were applied to fresh cut mangoes previously inoculated with E. coli, Salmonella typhimurium, Staphylococcus aureus and L. monocytogenes, inhibiting them, without affecting the characteristics of the fruit, according to the sensory evaluations.
4.2. Microencapsulation techniques
To ensure that phenolic compounds remain stable without altering their composition and beneficial properties, from extraction to consumption, it is necessary to prevent their deterioration by applying technologies or techniques such as microencapsulation, which has recently been used for the protection of phenolic extract of mango peel and seed (Table 3). Ribeiro et al. (2020) mention that this technique protects the compounds by incorporating them into an ideal matrix, preserving qualities, functionalities and with the opportunity to improve them.
In addition, Pattnaik et al. (2021) mention that it the advantage of controlling its release in the gastrointestinal tract so that the consumer can take full advantage of its properties, which is indispensable today.
The most commonly employed process is spray drying for its simplicity. According to Ozkan et al. (2019), this continuous physical-mechanical process is based on feeding the compound with the coating polymer in the dryer, they are mixed and then, inside a chamber provided with hot air, upon contact, the particles evaporate, dry, are separated by a cyclone and are collected as powder, which is affected by parameters such as feed flow, type and percentage of the coating material, temperature and flow of the drying air, among others. Another microencapsulation method widely used due to the use of low temperatures is freeze-drying, which consists of freezing (< 0 ºC) of the emulsion, subsequent sublimation, by means of vacuum pressure to finally obtain powder microcapsules, with low moisture level and high porosity due to the slow freezing process (Pattnaik et al., 2021). Another physical method is extrusion, which consists of passing the liquid solution formed between the compound and the encapsulating material through an orifice, and as a result of gravity, surface tension, momentum and friction, droplets are formed and then dried. The size of the powder is mainly affected by the diameter of the orifice (Ribeiro et al., 2020).
Regarding the physicochemical processes of micro-encapsulation of polyphenols from mango peel and seed, according to the literature, methods such as microencapsulation with ultrasound have also been employed. Pattnaik et al. (2021) mention that, due to the sound waves, bubbles are generated and grow at the food/polymer emulsion interface. Subsequently, the low-frequency acoustic intensity causes shearing, which results in the breaking of the emulsion, forming smaller particles.
There are other techniques that have also been widely used for the microencapsulation of phenols from various raw materials and also other bioactive compounds. Some, based on Ozkan et al. (2019); Ribeiro et al. (2020) are physical processes such as fluidized bed, interfacial polymerization and coacervation (simple or complex), chemical processes such as co-crystallization, ionic gelation, double emulsion and supercritical fluids, in addition to physicochemical ones such as liposome formation and molecular inclusion.
Regardless of the process, an important factor is the selection of the coating material, which has a direct influence on the performance of the microcapsules, their characteristics and subsequent release (Lim et al., 2019). It is essential that the encapsulant must provide emulsifying properties, be biodegradable, resistant to the intestinal tract, have low viscosity and hygroscopicity, provide maximum protection to the nucleus against adverse conditions, without being reactive with it, lack flavor and have economic viability, in order to be suitable in the food field. There are many coating agents, Ozkan et al. (2019) emphasize polysaccharides such as starches, maltodextrins, chitosan, gums, agar, cellulose and alginate, proteins such as gelatin and albumin, but lipids such as wax, paraffins, lecithin and glycerides are also used.
When choosing the ideal microencapsulation technique, factors such as the complexity of the method, the cost and whether it can be done continuously or discontinuously according to our requirements should also be considered.
Table 3 Recent research on the microencapsulation of phenolic extract from mango peel and seed
By-product/mango variety | Type of process/coating material | Optimal process parameters | Main findings | References |
Peel/V.N.S. | Ultrasound/maltodextrin and whey protein concentrate (20:80) | t: 1 h, T: 50-60 ºC; CM: 10%, P: 160 W; f: 20 kHz | 303.4 nm particle size, with a Zeta potential* of -24.76 V and 80.99% encapsulation efficiency | El-Messery et al. (2021) |
Seed/V.N.S. | Spray-drying/maltodextrin | Tai: 150 ºC; CM: 10%; Φ: 25 g/min; p: 0.1 MN/m2 | 127 mg GAE/g and 217,700 mmol TE/100 g DPPH, 0.32 g/cm3 bulk density, 3.17% moisture and 88.75% water solubility index and a yield >60%. | Lim et al. (2019) |
Seed/V.N.S. | Spray-drying/maltodextrin | Tai: 150 ºC; CM: 8%; p: 0.1 MPa | 3.56% moisture, 2.58% water absorption rate, 96.14 mg GAE/g, 129.46 mmoL ET/g DPPH, 24.28% maximum water solubility index plus acceptable color | Siacor et al. (2020) |
Peel/Ataulfo | Ultrasound-assisted polymeric double emulsion /Tween 20 | t: 4 min; T: environmental; f: 3000 rpm; emulsion: 25% water/oil and 75% pure water; A: 100 µm | 98.65 encapsulation efficiency, in addition to high trans-resveratrol recovery (97.84%), particle size (3.68 µm) and viscosity (11.06 mPa-S). Despite this, it showed instability during storage for 21 days. | Velderrain-Rodríguez et al. (2019) |
V.N.S.: variety not specified; t: time; Tai: air inlet temperature; T: temperature; CM: concentration of the coating material; f: frequency; p: pressure; Φ: feed flow rate; p: pressure; A: amplitude
*A measure of attraction or repulsion between microparticles, which directly determines their stability.
Based on information from Ozkan et al. (2019); Ribeiro et al. (2020), Figure 5 shows that the level of complexity is proportionally direct to the cost of processing, but in addition, the same relationship can be considered with respect to the type of production which can be discontinuous (g/h), semi-continuous (kg/h) or continuous (ton/h). For example, spray drying can be used or adapted without much difficult to a continuous scale, fluidized bed to a semi-continuous scale and ionic gelation to a discontinuous scale.
5. Current and future challenges
According to the various studies conducted on the phenolic potential of mango peel and seed and the properties it confers on them, they are a promising ingredient that could be used for pharmacological and food purposes (Ashoush & Gadallah, 2021). Their use would also allow a circular economy (Alañón et al., 2021) that, in addition to replacing the use of synthetic additives, which often have negative impacts on health (Pal & Jadeja, 2020), would reduce chemical and microbiological contamination, resulting from the decomposition of by-products. However, it is necessary to deepen in their properties to know the different effects they can cause when interacting with the food matrix, in contact with other compounds, in different doses and the type of benefit to be obtained; for example, considering the antimicrobial effect, in the elaboration of yogurt in the study of Pérez-Chabela et al. (2021), only pathogenic bacteria and other fungi and yeasts were inhibited, allowing the growth of lactic acid bacteria, which was ideal for fermentation, positively influencing the final quality of the product.
Regarding the extraction and microencapsulation methods, the different variables involved in the process should be thoroughly investigated in order to improve them, achieving optimal levels of polyphenols and antioxidant activity, as well as increasing bioavailability in the gastrointestinal digestion stage. In the research of Pattnaik et al. (2021), a precise analysis of these processes was carried out. They especially emphasized the use of emerging non-thermal technologies to avoid degradation of the compounds and, the use of safe solvents during extraction, such as CO2, water or deep eutectics. It is also advisable to analyze the use of combined techniques to optimize the process and try to apply it on a large scale, a promising challenge for the food field.
6. Conclusions
Mango peel and seed contain a large amount and variety of phenolic compounds with a high antioxidant and antimicrobial activity. These qualities can be exploited especially for their potential health benefit, in addition to contributing to a circular economy and to the reduction of environmental pollution generated by the decomposition of the aforementioned by-products.
It was evidenced by research their mechanism of action antimicrobial by the chemical interaction that causes various effects on the structure of the bacterial and fungal cell membrane, up to causing death, and antioxidant by stabilizing free radicals, chelation of metals and inactivation of enzymes, important factors in food preservation and also in the prevention of several diseases, especially chronic. Moreover, the various applications of phenolic compounds in the food industry were determined, standing out as a functional ingredient due to their capacity to influence the sensory and physicochemical characteristics of the foods, without affecting their quality.
Finally, as processing technologies, concerning extraction and microencapsulation, there are a wide variety of methods; therefore, in order to obtain better results, it is necessary to improve them and experiment with the use of combined techniques, taking into account that they are environmentally friendly.