1. Introduction
Pear (Pyrus communis L.) orchards in Chile occupy approximately 8,800 ha, where 2,200 ha (25%) are cultivated in the O’Higgins region (ODEPA, 2018). At the world level, the pear production reaches ~27 million tons, where the main producers are China, USA, Argentina, and Italy (FAOSTAT, 2018). During the 2018/2019 season, Chile exported near 25 million boxes of pears (ODEPA, 2020). The main pear variety is Packham´s Triumph with approximately 3,000 ha. Despite its economic significance, 54% of Chilean orchards are more than 20-years old, with plant densities varying from 300 to 3,000 plants ha-1. Pears cultivated in Chile are mainly exported to North America (~12,000 t), Latin America (~60,000 t), and Europe (~72,000 t) (Universidad de Talca, 2018). In low-density pear orchards, when the decay process begins, the usual recommendation is to replace affected plants, because the low productivity in one plant would represent about 0.2% to 0.3% yield loss per hectare.
Orchard decay is a problem that seriously affects the production, growth, and duration of pear trees. It consists of the systematic loss of root mass, resulting in a severe reduction of the aerial part and yield reduction. In the case of pear trees, decay is a problem directly related to the decrease of rhizogenesis, which is produced by many chemical and physical soil factors such as mechanical impedance by compaction (Brunetto et al., 2015; Morales-Olmedo et al., 2015). Furthermore, factors as pear root wooly aphid, nematodes, excess or deficit or quality of water irrigation, loss of soil structure, caused by the traffic of agriculture machinery, and the abnormal patterns in the water movement in the soil, are considered important factors causing decay in pear orchards (Brunetto et al., 2015; Ling-Fei et al., 2013; Poblete et al., 2013). Excessive water supply, high water table, and low porosity reduce the root growth (Jackson, 2003). On the other hand, soil moisture deficit affects primary root growth and its spatial distribution.
Soil temperature and nutrition are factors that also affect root growth. Pear roots begin growing at a temperature of 7-8 °C. Freeze damage could occur in superficial roots if air temperature reaches -9 °C. The nutrient demand of fruit species is dependent on the biomass produced by the different organs and mainly on fruit production; the total includes 3-4 kg N ton-1, 0.5 kg P ton-1 and 1.5 kg K ton-1; corresponding to 30-70 kg N ha-1, 20-30 kg P2O5 ha-1 and 70-100 kg K2O ha-1, depending upon yield and soil type.
To handle these issues, the concept of Integrated Nutrition Management (INM) has emerged as a strategy used to improve soil properties and plant quality. It combines organic matter (OM) application (as a source of carbon, nutrients, microorganisms, and organic substances) with chemical fertilization to stimulate rhizogenesis for improving plant nutrition and soil quality (Roussos et al., 2019; Das et al., 2015; Ortega, 2015; Wu & Ma, 2015). The use of INM has resulted in significant increments in soluble C, root density, root dry weight, and plant biomass in pear, table grape, nuts, apple, and cherry trees (Martínez et al., 2018; Flores et al., 2015; Ortega, 2015). Besides, important soil effects as the increase in soluble P, available N, and β-glucosidase activity occur through an improved OM mineralization process (Roussos, 2019; Fincheira-Robles et al., 2018; Sorrenti et al., 2012; Burg et al., 2011). To evaluate the effects of the application of an INM program, different soil properties must be measured and correlated with agronomical indicators like vigor by normalized difference vegetation index (NDVI). Besides, bulk density, soil porosity, or soil enzymatic activities are important indicators of the influence of management systems on crops (Ouyang et al., 2018; Nimmo, 2013; Martínez et al., 2010). Many soil processes are influenced by soil biota, which releases enzymes (i.e β-glucosidase and phosphatases) that participate in mineralization processes and solubilization of nutrients (i.e organic P) (Merino et al., 2016; Kujur & Kumar Patel, 2014; Riches et al., 2013). Furthermore, plant tissue analysis must be performed to determine potential nutrient deficiencies or excess to perform follow up of the fertilization management (Shunfeng et al., 2018; Ikinci et al., 2014).
The objective of this research was to evaluate INM as an agronomic strategy to recover a low-density, decayed pear orchard. The focus of the study was to evaluate the effects of INM on: (1) soil quality, (2) plant vigor and nutritional status (3) spatial variability of soil properties, and (4) yield and SOM changes.
2. Materials and methods
2.1 Location
The experiment was performed in Peumo commune (Rapel Valley, Chile) located at the coordinates 34(19’34.92’’ South and 71(15’23.67’’ West. The study area corresponds to a semi-arid, Mediterranean region, with a temperate climate. The soil belongs to the order Mollisol, which presents a silt loam texture, neutral pH, low organic matter content (~2%), and medium fertility. Temperatures are in the range of 5.5 to 27.6 ºC, while precipitation varies between 400 and 420 mm yr-1.
2.2 Plant material and growth conditions
The pear orchard (Pyrus communis L), Packam’s Triumph variety (on winter Nellis rootstock) was established in July 1976 in low density (333 plants ha-1). The planting density was 6 x 4.6 m. No organic matter was applied at planting. Pruning material was chipped and left between rows, every season, for the last sixteen years. The orchard was drip-irrigated with a double drip line with 6 drippers per plant (0.8 mm ha-1 hour-1). The average yield of the orchard was 60 ton ha-1, 75% exporting quality. The orchard presented a large spatial variability in terms of aboveground biomass due to decayed plants characterized by lower shoot growth, smaller size, and chlorotic leaves, compared to healthy plants.
2.3 Organic amendment and chemical fertilization
Mature compost type A, according to Chilean standard (NCh 2880/04-2016), was produced from grape pomace. The material had 20% of C, a NH4-N /NO3-N/ ratio of 0.81, and low content of trace elements (INN, 2016) (Table 1). The compost was applied in a band around each tree, during wintertime (July) of each evaluated season. The chemical fertilization contained N, P2O5, and K2O and it was applied during the growing season from October to February.
2.4 Experimental design
To evaluate the effects of INM two treatments were applied: T1: control (conventional) and T2: integrated nutrition management (INM). Treatments were assigned on 189 pear trees of different initial vigor (very low, low, medium, and normal). The initial vigor was determined by an existing aerial NDVI image. The experimental design was completely randomized, in a factorial arrangement of 2 treatments x 4 vigor levels, with four replicates. The INM treatment consisted of the application of 4 ton ha-1 of carbon (C) as compost, together with an adjusted chemical fertilization of 70 kg N ha-1, 20 kg P2O5 ha-1, and 66 kg K2O ha-1 season-1, respectively. The control included only the chemical fertilization at the same rates. The irrigation water applied was, on the average, 9.200 m3 ha-1 season-1, corresponding to 27.6 m3 plant -1 season-1. The management for recovery also included the absence of winter pruning in the first season, pruning in the second one, and removal of all fruit set in summer each season.
2.5 Evaluation of agronomic variables
For each pear tree, the total growth of branches ≥ 20 cm was measured. Four branches (one at each cardinal point), at 1,5 m height, were selected for NDVI measurement. The NDVI was measured using the active sensor Crop Circle ASC-210 (Holland Scientific®); 20 leaf samples per tree were taken at different heights. Root density was determined using a known volume of soil which was washed through a 2-mm sieve for preventing the passage of the roots. Collected roots were carefully washed and dried in an oven with forced air at 65 °C. Root density was reported in kg root m-3 of soil. Pruning weight was determined from each tree in winter (g tree-1).
2.6 Tissue analysis
The foliar analysis was performed in the summer, following the methodologies proposed by the National Laboratory Accreditation Commission (Sadzawka et al., 2007).
2.7 Soil analysis
Soil pH and electrical conductivity (EC) were measured according to Sadzawka (1990). Ammonium (NH4-N) and nitrate (NO3-N) contents were quantified by the colorimetric method described by Mulvaney (1996). Phosphorus availability was estimated by using the NaHCO3 extractant and determined according to Sadzawka et al. (2006). Organic matter content was determined by the Walkley and Black method (Sadzawka et al., 2006). Yeast and phosphate solubilizing microorganisms were grown in potato dextrose agar and SMRS1 agar, respectively (Novo et al., 2015). Microbial colonies were counted as colony forming units per fresh gram of soil (CFU g-1) (Novo et al., 2015). Furthermore, β-glucosidase was evaluated by measuring the p-nitrophenol released from p-nitrophenyl- β-D-glucopyranoside (pNPG), expressing the results in terms of β-glucosidase units (UBG = µg p-nitrophenol glucopyranose released g1 h-1 of the dry sample).
2.8 Soil quality and yield follow up after 5 growing seasons
After five growing seasons, a systematic soil sampling was performed at 30 cm depth to evaluate the changes produced with the application of INM. A total of 20 composited samples (5 probes point-1) were collected in a systematic grid, 10 of them for the INM and the remaining 10 for the conventional one. Each sample was georeferenced using a Global Navigation Satellite System (GNSS) unit. The following variables were measured: soil pH, soil organic matter, soluble C, and dehydrogenase activity, using the methodologies described before. Along with that, the yields for the pear orchard were recorded for the whole field.
2.9 Statistical analyses
Data were evaluated by correlation and regression analyses, and independent sample t-test using the Stata ® software. The stepwise procedure was developed to estimate a regression model to explain root density. Properties measured after five years, were mapped for the whole field, to evaluate their spatial variability. Maps were performed using the kriging interpolation algorithm in the software SMS (AgLeader Technology, Ames, Iowa, USA).
3. Results and discussion
Pear is one of the most important fruits worldwide (FAOSTAT, 2018). After some years of production, orchards show a decay process, which affects tree growth and production, caused by several physical, chemical, and biological changes in soil properties, as soil infiltration, water holding capacity, electrical conductivity among others (Aruani et al., 2012). Using the tools of integrated nutrient management (INM), through the application of organic matter and adjusted mineral fertilization it is possible to improve soil quality, nutritional status, and root development to increasing tree vigor, rhizogenesis and yield (Martínez et al., 2018). The changes in soil properties could be “tracked” using sensible indicators as biological activity or potential enzymatic activity, which are soil quality indicators due to its participation in organic matter degradation and mineralization (Huera-Lucero et al., 2020; Adetunji et al., 2017, Angulo et al., 2014). The degradation of compost and other cellulose-related compounds in soil is directly related to potential of endoglucanases, especially to β-glucosidase, which is a very practical and sensitive indicator (Partey et al., 2019; Martínez et al., 2018). Other studies evidenced that vermicompost, straw mulch, farmyard manure applied to a subtropical mango (Mangifera indica L.) orchard improves microbiological activity, soil nutrient availability and carbon stock, enhancing fruit yield (Kumari et al., 2020). In this study, potential β-glucosidase activity increased 66% under INM treatment at all evaluated vigor levels (478.0 µg p-nitrophenol g-1h-1 versus 288.3 of control µg p-nitrophenol g-1h-1) (Data not shown). The values of β-glucosidase are considerably higher compared to previous studies in table grape soils (~ 50 μg p-nitrophenol g-1h-1), suggesting differences in soil quality, irrigation, and fertilization management (Morugán-Coronado et al., 2019; Martínez et al., 2018). Moreover, the data support that β-glucosidase activity increased under INM due to the presence of organic amendments, which improve the microorganism’s activity (Günal et al., 2018). Our results were consistent with those obtained by Mariscal-Sancho et al. (2018), who concluded that β-glucosidase activity depends directly on the presence of organic carbon and biological activity, constituting an indicator of soil quality. Furthermore, the increase in β-glucosidase activity indicates further degradation of cellulose converted into glucose, which is an energy source for soil microorganisms, thus increasing the activity of the microbial biomass (Partey et al., 2019; Martínez et al., 2010).
Regarding physical properties, INM reduced soil bulk density (BD) compared to the control treatment, regardless of the vigor level (0.8 vs 1.1 g cm-3) (Data not shown). BD impacted the porosity, which increased by 19% under INM (55.9 to 66.6 % porosity). These results showed that BD decreased under INM, suggesting its positive influence on root development and nutrient uptake. Besides, BD determines the pore space, which is a key property for irrigation and soil aeration processes. On the contrary, a high BD generally indicates compaction problems, decreased porosity, poor aeration, reduction of water infiltration rate, and a mechanical impediment for root growth (Martínez et al., 2018). It is noted that organic matter included in INM treatment can play an important role to modulate BD (Chen et al., 2017; Liu et al., 2013).
Additionally, root density significantly increased with INM application, reaching around 20 kg cm-3 versus 2.5 kg cm-3 of the control treatment (Figure 1). It noteworthy that root density is a relevant indicator of rhizogenesis process. Root density was almost 1,000% larger under the INM treatment compared to conventional management. Similar results were obtained by Baldi et al. (2010ab) using annual compost applications, increasing root density by 435% and the SOC from 2.45 to 7.82%. Martínez et al. (2018) found an increased root density with the increase in C rate in a study performed in table grapes because of the effects of different organic matter fractions on BD and the improvement of porosity. Moreover, the presence of organic matter in the soil increases biological and microbiological activity, improving nutrient availability and releasing growth-promoting substances into the soil solution that improves root development (Morugán- Coronado, 2019; Sharma et al., 2017; Magdoff & Van Es, 2010). A larger root mass improves water and nutrient uptake, stimulating the rhizogenic process and microbial - plant beneficial associations. Besides, enhanced root growth also improves plant greenness and aboveground biomass (Roussos et al., 2019; Wasaya et al., 2018; Ortega & Fernandez, 2007). It has been shown that compost application produces larger shoot length and diameter compared with mineral fertilization in apple and pears (Safaei Khorram et al., 2018). In this study, a significant increase in growth parameters as shoot growth and pruning weight was found (Data not shown).
Few significant effects were observed on foliar nutrient levels. The INM increased Cu and Na concentrations in leaves compared to conventional treatment (P < 0.05). Besides, Zn concentration was 25% lower under the INM treatment, probably by Cu competition. Significant differences were observed in foliar P levels, with a signifi cant orderly interaction treatment x vigor. On average, P levels were lower under INM compared to conventional management. Probably, the dilution effect could explain part of these results. Nevertheless, no statistically significant differences were found in the leaf content of N, K, Ca, Mg, Mn, Fe, and B (data not shown). A nutritional index (NI) was built to estimate absolute amounts of nutrients within the tree biomass by multiplying nutrient concentration times NDVI. This index allows comparing the nutrient amounts present in the leaves eliminating the concentration/dilution effects. Specifically, NDVI corre sponds to a non-linear combination of the visible (red) and near-infrared bands to enhance vegetation (Tan et al., 2020; Xue & Su, 2017). Table 2 shows nutrient amounts in leaves as estimated by NI. The INM had a significant effect on NI compared to the conventional treatment. The INM affected nutrient absorption by plants and stimulated plant development (Ortega & Fernandez, 2007). On aver age, NDVI measured in summer was almost 17% larger for INM compared to the conventional treatment. The INM treatment increased nutrient uptake as compared to the conventional treatment in terms of N (13.6%), K (17.6%), Ca (11.4%), Mg (11%), Cu (41%) and Fe (23%). On the other hand, NI for Zn was 13.2% lower under INM corroborating that the decrease was not due to the dilution effect. The results showed significant effects of INM on Cu leaf con tent since the field had a high copper content (>50 ppm DTPA-Cu, data not shown). Consequently, Zn content was 25% lower under INM, probably by Cu competition. The large increase in Cu could be explained due to its high content in the soil as explained above. Previous studies indicate that the INM, and particularly the applications of organic matter, generates changes in the absorption ratios of metals such as Cu or Zn (Salam et al., 2019). In the case of P, although there were significant statistical differences in foliar levels for INM respect to conventional management, there was significant interaction treatment × vigor. On average, P foliar levels were lower under INM compared to conventional management. However, the difference was larger as the plant vigor (biomass) increased. This is an expected result since under INM a dilution of P content with vigor was observed. In the case of N and K results differ from those obtained by Ortega & Fernandez (2007), who found statistical differences in the uptake of N, P and K in ryegrass.
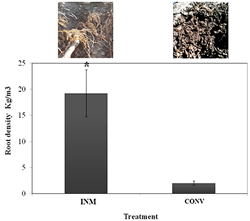
Figure 1 Effect of INM on root density in pear after 2 seasons. * = Indicates statistically significant differences. Abbreviations: INM= Integrated Nutrition Management, CONV= Conventional.
Table 2 Treatment and vigor effects on Nutritional Indices (NI). Abbreviations: CONV =Conventional, INM= Integrated Nutrition Management, AV= Average
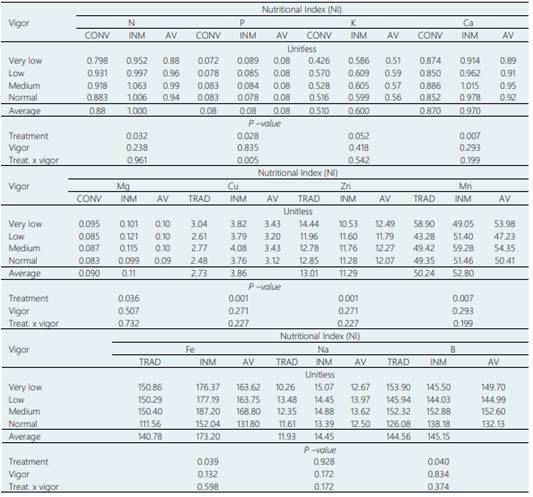
Additionally, we evaluated the INM to determine its impact in soil properties after two seasons. Soil samples corresponding to INM and conventional treatments were collected after two seasons from application to evaluate changes in chemical and biological properties (Table 3). The INM increased (P < 0.05) electric conductivity (0.28 versus 0.17 dS m-1, respectively), organic matter (5.25 versus 2.39 %, respectively), and available nitrogen (57.7 versus 3.9 ppm, respectively) compared to the conventional treatment. Regarding biological activity, there were statistical differences in β- glucosidase activity with higher potential activity for the INM treatment (316.8 vs 132.5 μg p-nitrophenol g-1h-1). The soil analyses are in concordance with the results of other authors (Partey et al., 2019; Mariscal-Sancho et al., 2018; Adetunji et al., 2017; Van Schoor et al., 2012). For soil nutrient contents, INM showed significantly higher levels than the control in terms of available N, NO3-N, and NaHCO3-P, similarly, as described by Riches et al. (2013) and Roussos et al. (2019).
To evaluate the more permanent effects of INM, soils were analyzed after 5 years from establishment (Figure 2). The results showed that after 5 years, there was no difference in soil pH of both evaluated treatments. It was possible to observe a large increase in soluble C and dehydrogenase activity, both properties related to rhizogenesis and biological activity in the soil. Besides, the application of mature and stabilized organic matter as compost improved soil organic carbon, nutrient content, plant available water and microorganism’s activity (Kranz et al., 2020; Sánchez-Monedero et al., 2019; Kallenbach et al., 2016; Angulo et al., 2014). Organic matter contents were higher in soil under INM compared to conventional management (SOM~ 8% versus ~ 5.5%). Besides, soluble C (35 mg Kg-1 versus 15 mg Kg-1) and dehydrogenase activity increased under INM (110 µg TFP g-1 day-1 versus 56 TFP g-1 day). The importance of the application of enriched compost with an adjusted inorganic fertilizer has been evidenced in rice crops through enhancing Zn, Cu, Fe, Ni, and Mn in soil and mitigating the Al toxicity in acids soil after ten years of application (Patra et al., 2021). Furthermore, Triverdi et al. (2020) reported that 60 years of INM with adjusted inorganic fertilizers improved carbon sequestration in corn (Zea mays L.) and corn (Triticum aestivum L.) crops at different soil layers (15-60 cm of soil depth). On the other hand, it has been found that the application of organic and inorganic amendments together with microbial inoculants improved P mobilization and solubilization at long- term cereal-vegetables- legume rotations (Ghosh et al., 2021). Figure 3 shows the spatial variability of soil pH, OM, soluble carbon, and dehydrogenase between treatments, which is reflected by color intensity.
According to the results obtained in this study, the recovery of decayed trees in a pear orchard must be performed from a holistic perspective. The orchard management recognizes a system where soil, plants and microorganisms coexist and interact with each other. An integrated nutrition management program allows the orchard recovery through the improvement of the physical, chemical, and biological soil properties directly involved in root growth. This was demonstrated by the regression model obtained using the stepwise procedure to explain root density (equation 1).
Where Root density: root density (kg/m3); β Glu: beta-glucosidase activity (μg p-nitrophenol g-1 h-1); EC: electrical conductivity (dS/m); pH: soil pH (pH units); OM: organic matter (%).
Table 3 Treatment effects on soil properties determined in spring after two seasons. Abbreviations: INM= Integrated Nutrition Management, CONV= Conventional Management
Variable | Unit | CONV | INM | Average | P-value |
pH | - | 7.4 | 7.3 | 7.4 | 0.52 |
Electrical conductivity | dSm-1 | 0.1 | 0.2 | 0.2 | < 0.01 |
Organic matter | % | 2.3 | 5.2 | 3.8 | < 0.01 |
Available nitrogen | ppm | 3.9 | 57.7 | 30.8 | < 0.01 |
NO3-N | ppm | 3.5 | 56.7 | 30.1 | < 0.01 |
NH4-N | ppm | 0.3 | 0.9 | 0.6 | 0.239 |
P-Olsen | ppm | 47.5 | 103.0 | 75.2 | 0.01 |
β-Glucosidase | μg p-nitrophenol g-1h-1 | 132.5 | 316.7 | 224.6 | < 0.01 |
Yeast count | UFC g-1 | 87.0 | 277.5 | 182.2 | 0.080 |
Phosphate solubilizers | UFC g-1 | 110.0 | 29.5 | 69.7 | 0.340 |
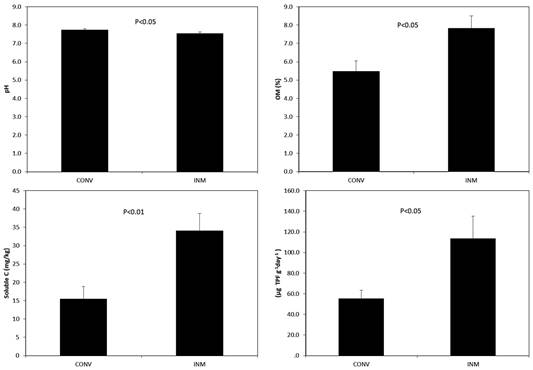
Figure 2 Comparison between conventional and integrated nutrition management in terms of soil pH (left above), organic matter (right above), soluble C (left below), and dehydrogenase activity (right below) after 5 years.
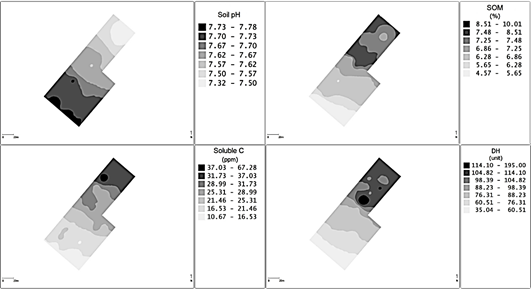
Figure 3 Spatial variability of soil pH (left above), organic matter (right above), soluble C (left below), and dehydrogenase activity (right below) after 5 years. In each map, the INM corresponds to the upper half of the field.
The model clearly describes the importance of soil organic matter and microbial activity on pear root density. Finally, Figure 4 shows the increase in pear yield in parallel to SOM increment. In terms of soil quality, several indicators significantly changed after 5 years of INM, which directly related to orchard productivity.
4. Conclusions
The main effects of Integrated Nutrition Management (INM) were an increase in soil organic matter and overall soil fertility, which increased microbial activity in the soil, decreased soil bulk density, and increased porosity, which in turn allowed for a better root development, which expressed in terms of improved vigor and a continuous increase in yield. Integrated nutrition management, along with proper canopy management, resulted in an effective practice to recover decayed pear orchards within an appropriate time frame. Although the integrated management of soils and crops is an ancient concept, demonstrating its effects in time and space will allow for a better use of organic matter and mineral fertilization, in both organic and conventional fruit agroecosystems, according to their site-specific requirements and objectives.