1. Introduction
The establishment of the biodiesel industry in Latin America, especially in Colombia, has generated an increase in the availability of crude glycerol (CGL) as a co-product, representing approximately 10% of the total biodiesel production (Colmenares-Quintero et al., 2020). The main component of CGL is glycerol, a sugar alcohol with gluconeogenic properties (Kholif, 2019). One of the main nutritional characteristics of glycerol is its high energy content, which is comparable to that of corn grain (Kupczyński et al., 2020). A previous study evaluated glycerol supplementation during different stages of lactation (Ogborn, 2006); glycerol is mainly used as an energy supplement (Bodarski et al., 2005) or as a substitute for corn and barley meal (Gaillard et al., 2018; Vito et al., 2018).
Studies carried out to date on glycerol supplementation have primarily been conducted with feeding systems containing total mixed rations (TMR). Accordingly, only few studies have explored the effects of glycerol supplementation on grazing dairy cows. According to Santana Júnior et al. (2013), glycerol supplementation in lactating dairy cows in tropical pasture (Brachiaria brizantha) conditions do not affect productive parameters, such as protein, lactose, total solids, non-fat dry extract, and milk urea nitrogen. The Kikuyu grass (Pennisetum clandestinum Hochst. Ex Chiov) is a cool-season perennial that is commonly used globally as grass for specialized dairy cattle herds in tropical climates (Fonseca et al., 2016). Kikuyu grass is characterized by a high concentration of both fiber and crude protein, mainly in a non-protein nitrogen form, and a low content of sodium and non-structural carbohydrates (Acero-Camelo et al., 2020). Studies on the potential of glycerol to improve milk production in cows grazing on perennial tropical grasses, such as Kikuyu grass, are currently lacking.
Combining the high energy content of glycerol and the nutritional profile of Kikuyu grass may be an important nutritional strategy to increase the competitiveness of dairy production systems on this type of pasture. Thus, we hypothesized that CGL supplementation increases yield without affecting the milk chemical composition of dairy cows grazing on Kikuyu grass. Therefore, the aim of this study was to determine the effects of different levels of CGL supplementation on dry matter intake, milk yield, milk protein yield, and milk fatty acid profile in dairy cows grazing on a Kikuyu-based pasture.
2. Materials and methods
2.1 Cows, dietary treatments, and experimental design
This study was carried out at the Paysandú experimental farm in Santa Elena (Medellin, Antioquia, Colombia). The farm is located at 6°12´25.2´´north and 75°30´08.83´´west at 2,579 m above sea level and has an average monthly temperature of 14 °C. All cow procedures were performed in accordance with the Animal Care and Use Committee of the Universidad Nacional de Colombia Medellín campus (protocol CEMED number 028).
Six multiparous Holstein cows in the first third of lactation were examined at 43 ± 10 days of lactation [average body weight (BW) of 559 ± 22.5 kg], with a mean milk yield of 26.8 ± 1.2 kg of milk/day. The cows grazed Kikuyu grass in a rotational stocking system with a 40-day resting period and one day per paddock at a stocking rate of 1.7 AU/ha. The cows were supplemented with an energy-protein concentrate (Table 1) at a rate of 1 kg DM/4.0 kg of milk. Supplementation was established and kept constant according to milk yield at the beginning of each period.
The cows were randomly distributed in a replicated 3 × 3 Latin square design. The following treatments were administered: CGL0 (control, no glycerol supplementation), CGL1 (addition of 750 g of CGL/cow/day), and CGL2 (addition of 1,500 g of CGL/cow/day). The experiment was carried out over 69 days (23 days per period), with 18 days of adaptation to glycerol supplementation and five sampling days within each period. CGL was obtained from a palm oil-based biodiesel production industry (Oleoflores GE, Codazzi, Cesar, Colombia) and contained 880 g/kg of glycerol, 80 g/kg of water, 40 g/kg of ash, and 8 g/kg of methanol. The estimated net energy of lactation (NEL) was 2.02 Mcal/kg of DM according to the NRC (2001).
Table 1 Ingredients and basal diet chemical composition
Kikuyu grass a | Concentrate | |
Ingredients (g/kg DM) | ||
Ground corn | - | 314.0 |
Soybean meal | - | 150.0 |
Cotton seed | - | 65.0 |
Sugarcane molasses | - | 50.0 |
Wheat bran | - | 404.0 |
Fish meal | - | 10.0 |
Premixb | - | 2.5 |
Calcium bicarbonate | - | 2.5 |
Magnesium oxide | - | 2.0 |
Chemical composition (g/kg DM) | Mean ± SD | Mean ± SD |
DM | 171.7 ± 1.6 | 901.1 ± 1.1 |
CP | 187.0 ± 2.4 | 193.0 ± 0.7 |
NDF | 639 ± 1.8 | 328 ± 1.3 |
ADF | 263 ± 1.9 | 161 ± 1.3 |
Lignin | 40 ± 0.7 | 22 ± 0.3 |
EE | 25.0 ± 0.5 | 41.0 ± 0.2 |
Ash | 101 ± 1.1 | 64 ± 0.4 |
NEl (Mcal/kg of DM) c | 1.3 ± 0.2 | 1.9 ± 0.4 |
aPennisetum clandestinum Hochst. Ex Chiov grass with a 40-day rest period b Vitamin and mineral premix contained (g/1000 g): vit. A, 5000 IU; vit. D3, 1,500 IU; Vit. E, 4 IU; Fe, 25 mg; Cu, 5 mg; Mn, 20 mg; Zn, 20 mg; I, 0.3 mg; Co, 0.05 mg; Antioxidant BHT, 60 mg; excipients C.S.P., 2.0 g.
c NEl: Net energy of lactation estimated based on NRC (2001).
2.2 Intake and Milk yield measurements
Milk yield was automatically measured during the daily milkings (05:00 and 17:00 h), and the total amount of CGL was divided into equivalent quantities during the day and manually mixed with the commercial concentrate during each milking. On the five sampling days of each period, samples of the concentrate and grass simulating selective grazing were obtained according to the "hand plucking" technique described by De Vries (1995). The samples were dried for 72 h at a temperature of 60 °C in a forced ventilation oven and ground to 1 mm to obtain a composite sample per cow per period. Grass dry matter intake was calculated from total fecal excretion and feed indigestibility. Total fecal excretion was estimated from the fiber mordanted with chromium (Cr-mordanted: 1.3 ± 0.2% Cr concentration) over 23 days, which corresponds with the duration of each experimental period (18 days of adaptation and 5 days of sample period), according to the methodology described by Udén et al. (1980). According to the average BW of the animals within each treatment, 46 g/cow/day of Cr-mordanted was administered in paper capsules with the concentrate, dividing Cr-mordanted into equal amounts at the daily milkings. Fecal samples from each cow were collected from the rectum twice per day, frozen at -4 °C, dried in a forced ventilation oven at 60 °C for 72 h, and ground to 2.0 mm to form a composite sample per period per cow. To establish feed indigestibility, the concentration of indigestible neutral detergent fiber (iNDF) in the grass, concentrate, and fecal samples was determined according to Raffrenato et al. (2018).
During the sampling days within each period, at the end of each milking, the individual production was recorded (kg milk/cow/day), and the compositional quality and fatty acid profile of the milk were analyzed. Briefly, 100 mL of milk was obtained from each milking from a Waikato® collector, and both samples were homogenized and mixed to obtain a composite sample per cow per day, which was preserved with Bronopol® (2-Bromo-2- nitro-1,3-propanediol) and maintained at -4 °C until analysis.
2.3 Laboratory procedures and calculations
Chemical composition analysis was conducted for the grass, concentrate, and fecal samples. The ash, dry matter, nitrogen contents, concentrations of neutral detergent fiber (NDF), acid detergent fiber (ADF), lignin, and ether extract (EE) were determined as described by Valencia et al. (2020). For iNDF determination, approximately 1.0 g of each sample (grass, concentrate, and feces) was incubated in duplicate for 240 h in vitro, with re-inoculation every 120 h, according to the procedure described by Raffrenato et al. (2018). ANKOM F57 bags with a pore size of 25 μm and a Daisy II® incubator (ADII, Ankom Technology Corporation Fairport, NY, USA) were employed according to the methodology described by Goering & Van Soest (1970). After incubation, the bags were removed, washed homogeneously in a washing machine, and then dried in a forced ventilation oven at 60 °C for 72 h. The concentration of Cr in the feces was determined according to Holden et al. (1994) using an atomic absorption spectrophotometer (AA-680, Shimadzu®, Tokyo, Japan). Dry matter intake of the grass (DMIf) was estimated using the following equation presented by Velásquez et al. (2018):
where TFE is the total fecal excretion (kg DM/day) obtained using Cr-mordanted with a marker recovery rate of 99.5% (Udén et al. 1980), FEC is the fecal excretion from the concentrate (kg DM/day), and FI is the grass indigestibility (kg/kg DM).
The offered concentrate, CGL, and orts were weighed daily to calculate the total dry matter intake (total DM intake, kg/day). The net energy intake (NE intake, Mcal/day) was calculated by multiplying the total DM intake and the net energy of lactation (NE1; Mcal/day) of the grass, concentrate, and glycerol.
The milk yield corrected to 3.5% fat (3.5% FCM) was calculated using the equation described by Tyrrell & Reid (1965): FCM = 12.82*FP + 7.13*PTP + 0.323*MP, where FP is the fat yield in kg/day, PTP is the protein yield (kg/day), and MP is the milk yield (kg/day). The milk yield efficiency was calculated by dividing the MP by the DMI.
The contents of protein, fat, and non-fatty solids in milk were measured by ultrasonography using an ultrasonic milk analyzer (Lactoscan®, LAC-S, Boeco, Hamburg, Germany). The composition of fatty acids (FAs) in the milk, including CLA, was obtained from the lipid fraction, which was extracted by boiling with a detergent solution of sodium hexametaphosphate ((NaPO3)6) and Triton X-100 (C8H17C6H4 (OCH2CH2)nOH). Methyl esters were prepared from the extracted fat sample as described by Mojica-Rodríguez et al. (2019). The FAs were analyzed using a gas chromatograph (GC-2014, Shimadzu®, Tokyo, Japan), with an FID detector and a capillary column (100 m × 0.25 mm °0.2 μm Rt 2560 Restek®). The following oven temperature program was employed: 100 °C for 2 min and heating at 2 °C/min up to 240 °C for 25 min. The temperatures of the injection port and detector were 250 °C and 270 °C, respectively; the carrier gas velocity was 1 mL/min; the split ratio was 1/100; and the injection volume was 1 μL. Each peak in the chromatogram was identified and quantified via retention time comparison using methyl standards for FA quantification (NuChekprep, Elysian, MN, No. GLC-603), and specific standards for trans-11 C18:1 and CLA conjugated linoleic acid isomers cis-9, trans-11 and trans-10, cis-12 of CLA quantification (Nu-Chekprep, Elysian, MN). The percentage of each FA was calculated according to the procedure described by Dhiman et al. (1999). The results are expressed as g FA/100 g of fat.
2.4 Statistical analysis
The data were analyzed as a replicated 3×3 Latin square design using the MIXED procedure of the Statistical Analysis System (SAS) software (version 9.2). The CGL supplementation level was considered as a fixed effect, and the period and animals were considered as random effects. The statistical model is presented as follows:
where Yijkl is the value observed in the plot for the i-th treatment, the j-th Latin square, the k-th cow, and the l-th period; µ is the overall mean; αi is the effect of the i-th treatment; βj is the effect of the j-th Latin square; λk(j) is the effect of the k-th cow within the j-th Latin square, γl(j) is the effect of the lth period within the j-th Latin square, αβ(ij) is the effect of the interaction of the i-th treatment and the j-th Latin square; and εijkl is the residual error. The mean effects of the treatments were analyzed using linear and quadratic contrast tests corresponding to supplementation with CGL in the basal diet. Differences were considered significant at P ≤ 0.05.
3. Results and discussion
We hypothesized that CGL supplementation in lactating dairy cows grazing on Kikuyu grass could increase milk yield without affecting the milk nutritional profile. Herein, we evaluated two levels of CGL: 750 (CGL1) or 1,500 (CGL2) g/cow/day versus a control supplement without CGL (CGL0). CGL supplementation was found to have a positive linear effect on total dry matter and energy intake, which increased milk yield and milk protein content without changing the fatty acid profile of milk. Accordingly, our hypothesis was confirmed.
The grass dry matter intake (DMIf) was not affected by CGL supplementation (P > 0.05; Table 2); however, the total DM intake increased linearly (P = 0.02). This result was also found for the total intake energy, which was higher with 1,500 g of CGL compared to control treatment (CGL2; P = 0.01). Such findings indicate that CGL supplementation has an additive effect on the dry matter intake of the diet, allowing an increase in both total DMI and energy intake, especially when 1,500 g of CGL was supplemented.
Table 2 Mean and Standard error of the mean (SEM) of intake and milk yield of grazing dairy cows supplemented with different levels of crude glycerol
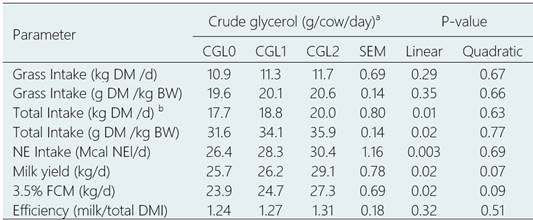
a CGL0, control without CGL; GL1, 750 g of CGL/cow/day; GL2, 1,500 g of CGL/cow/day. b Sum of grass dry matter intake, concentrate intake, and crude glycerol intake.
This result aligns with that of Fisher et al. (1971) and Bodarski et al. (2005), who supplemented cows in the first third of lactation with 500 mL and 470 g of glycerol, respectively, and found an increase in the total DMI of approximately 10% in TMR. By including glycerol in the diet, Bajramaj et al. (2017) also found an increase in DMI in dairy cows when 15% glycerol was added to a diet with 70% forage, thereby demonstrating the beneficial properties of glycerol on diet palatability and its appetite-stimulating effect. Thus, glycerol supplementation may be a useful nutritional strategy to increase DMI and energy intake in lactating dairy cows.
When Santana Júnior et al. (2013) assessed other conditions, no changes in the DMI of the grass were found when 0, 94, 191, 289, and 389 g of CGL/kg were employed as a replacement for corn grain in early lactating cows grazing Brachiaria brizantha, with approximately 14% and 36% crude protein and ADF, respectively. The difference in DMI responses to CGL supplementation between the study by Santana Júnior et al. (2013) and this study may be partially attributed to the nutritional grass profile. In this study, Kikuyu grass had a higher crude protein content (18.7%) and lower ADF (23%) than the Brachiaria brizantha used in the study by Santana Júnior et al. (2013). According to some reports, for diets whose predominant proportion is forage, glycerol may promote the efficiency of nutrient utilization in the rumen, thereby increasing the ingestion (Kholif, 2019).
Selecting an adequate amount of glycerol for use as a supplement is essential to enable the maximum potential of glycerol, particularly in lactating dairy cows grazing on perennial tropical grasses. This is because glycerol may negatively affect the adhesion of microorganisms to fiber and cellulolytic bacterial enzymatic activities, which ultimately reduce the extent of forage fiber degradation (van Cleef et al., 2018). Torres et al. (2021) concluded that the amount of glycerol in dairy cattle diets is a predominant factor. Accordingly, the inclusion of 10%-15% glycerol, either as a replacement or as part of DMI, does not lead to negative effects on the intake of the other fractions of the feed, which could occur due to the substitution effect generated by feeding with glycerol. Previous studies on grazing heifers did not report any negative effects on feed intake when up to 150 g/kg CGL was included in the DM diet (Facuri et al., 2014). In the present study, supplementation with 750 and 1,500 g of CGL represented approximately 4.0% and 7.5% of the total DMI, respectively. Further, these amounts were not found to have adverse effects on grass and concentrate DM intake. Therefore, glycerol supplementation up to 75 g/kg DMI may be indicated in lactating dairy cows grazing on perennial tropical grasses without inducing any negative effects on diet intake.
The milk yield and yield of fat-corrected milk (FCM 3.5%) showed a linear response, increasing by 13.3% and 14.2%, respectively, with the highest level of CGL supplementa tion compared to diet without the inclusion of CGL (CGL0; P = 0.02). However, there were no changes in milk yield per DM unit intake (P = 0.29). The increase in total DMI and energy intake led to an increase in milk yield and FCM with the addition of 1,500 g of CGL. Such findings indicate that the gluconeogenic effect of glycerol induces higher energy availability for milk yield, mainly from ruminal propionate (Kholif, 2019). Notably, this volatile fatty acid can participate in 30%-72% of glucose synthesis occurring in the liver tissue (Reynolds et al. 2003). Previously, by including 15% and 30% of CGL (83% glycerol) in diets with a roughage:concentrate ratio of 45:55 (DM basis), Ezequiel et al. (2015) found an increase in efficiency and no effect on milk yield and FCM when 30% of CGL was included in the diet. Such findings suggest that the efficiency can only be maintained by NEFA during the first 21 days postpartum. However, during longer lactation periods, the decrease in DMI could lead to a decrease in milk yield per unit of DM consumed.
The concentration of milk protein was 2.1% higher in CGL2 than in CGL0, which may be related to milk protein yield (P = 0.01; P = 0.01, respectively; Table 3). Such findings align with those of Kass et al. (2012) and Wilbert et al. (2013), who included up to 15% and 12% of CGL in the diet of cows during lactation, respectively. Notably, the increase in energy intake would lead to a lower use of amino acids for the synthesis of glucose, thereby enabling their use in protein synthesis in milk. Bajramaj et al. (2017) reported an increase in milk protein yield of 263 g/day in dairy cows during the first third of lactation when 15% glycerol was added to a diet with 70% forage. Similarly, several studies have suggested that the use of glycerol as a rapid fermentation compound would compensate for energy deficiency in the rumen, permitting a higher use of nitrogen by ruminal microorganisms with a reduction of ammonia nitrogen in the rumen, increased synthesis of microbial proteins, and a subsequent increase in the concentration and milk yield of proteins (Wang et al., 2009; Kass et al., 2012). Under the experimental conditions of this study, the high content of crude protein and non-protein nitrogen of Kikuyu grass, which enabled the fast energy supplied by CGL, may stimulate the synthesis of microbial protein, an important protein source for lactating grazing cows, ultimately allowing an increase in both the concentration and milk yield of protein.
Milk fat concentration and fat yield did not differ between groups of cows (P > 0.05). However, Thoh et al. (2017) reported a decrease in milk fat content when goats were fed 10% glycerol as part of their diet; this reduction was attributed to a lower molar proportion of ruminal acetate, which is the main precursor of milk fat. The fatty acid (FA) profile in milk, as well as the concentration of saturated fatty acids (SFAs), unsaturated fatty acids (UFAs), and the SFA:UFA ratio, were not affected by treatments (Table 3). The effect of glycerol supplementation on milk FA composition in grazing dairy cows has not been sufficiently evaluated. However, Ezequiel et al. (2015) found a linear increase in odd-chain FAs in milk with the addition of 15% and 30% CGL in the diet, suggesting an improvement in ruminal microbial synthesis with an increase in propionyl-CoA, a precursor in the synthesis of these FAs. Dietary glycerol was also found to increase the concentration of cis-9, trans-11 C18:2 (CLA) in milk; this increase might be due to the decrease in ruminal pH caused by the replacement of corn with glycerol. In contrast, Ariko et al. (2015) found an increase in odd-chain FAs in milk when 52, 104, and 156 g/kg of glycerol were included as replacements for barley meal in the DM of TMR diets for dairy cows in the first third of lactation. These researchers attributed the higher odd-chain FAs in milk to an increase in the ruminal propionate concentration. However, there were no decreases in the de novo synthesis of short-chain FAs in response to the increase in the concentration of butyrate in the rumen, which was positively correlated with the concentrations of C6:0, C8:0, and C10:1 cis9 in milk. Therefore, more research should be carried out to determine the effects of including crude glycerol in the synthesis of FAs with functional attributes, especially in dairy cows grazing on tropical pastures.
The concentration of both milk lactose and yield increased linearly with the inclusion of CGL (P = 0.004 and P = 0.01, respectively). Such finding may be due to the higher energy intake resulting from CGL2. Van Hoeij et al. (2017) reported that an increase in dietary energy intake has a positive effect on milk lactose concentration in cows. Although the concentration of total solids was not affected by CGL addition (P = 0.79), the yield of milk solids was 14.3% higher in the CGL2 group than in the CGL0 and CGL1 groups (P = 0.04). The higher milk solids observed in the CGL2 group were expected due to the higher milk lactose yield promoted by CGL2; this is because lactose is the main milk solid in cattle (Costa et al., 2019).
4. Conclusions
Supplementing grazing dairy cows with 1,500 g of CGL/cow/day did not induce any substitute effect on grass intake, but enabled higher total dry matter and energy intake, resulting in an increase in milk yield and protein milk content and no changes in the FA profile of milk. Thus, this co-product may be used as part of a nutritional strategy to increase the productivity of dairy production systems on tropical perennial pastures, such as Kikuyu grass. Future studies on the inclusion of crude glycerol and its effects on the synthesis of FAs with functional attributes, especially in dairy cows grazing tropical pastures, are necessary.