1. Introduction
3D printers are robotic machines that create objects through a process called "further manufacturing". There are four applicable techniques in food 3D printing, among them are material extrusion, selective laser sintering, hot air sintering, and liquid bonding, of which material extrusion provides greater versatility in terms of applicable ingredients (Godoi et al., 2016; Derossi et al., 2018; Severini et al., 2018; Mantihal et al., 2020; Jayaprakash et al., 2020). Accordingly, experts consider that extrusion-based techniques have the greatest potential for industrial scaling.
Most studies have focused on the development of edible inks for extrusion printing, which offers a much wider range of printable and edible materials. In extrusion-based printing, a motorized syringe extrudes food materials onto a platform and the object is built layer by layer based on a pre-designed digital model (Ma & Zhang, 2022). Food inks are often viscoelastic, to provide the necessary structural integrity while remaining extrudable through the print nozzle (Wilms et al., 2021). In some cases, additives such as hydrocolloids, starch, enzymes, and anti-browning agents are used. Likewise, chocolate, cheese, sugar, and starch-based materials are among the most used ingredients for 3D printing of foods; additionally, there is enormous interest from the food industries in the implementation of alternative raw materials based on meat, fruits, vegetables, insects, and algae (Escalante et al., 2021).
However, there are some limitations regarding its application in the food industry, since the structural and nutritional quality of the final product depends on the type of material used and the selected printing technique, which is why research is still being carried out in this field. (Zhang et al., 2021; Derossi et al., 2021).
This article reviews the use of bioprinters as an alternative for food production, as well as conceptual and ethical aspects, advantages and disadvantages, and their applications.
2. Basic concepts of bioprinting
3D printers are robotic machines that create objects using a process also called "additional manufacturing." This means that instead of defining the shape of an object by removing leftover raw material, these machines only use the necessary material in the form of liquid, powder, or filaments which are then melted or solidified to create the final shape. With 3D printing, a machine can generate the most complex objects following the instructions of a 3D digital model designed with CAD (Sher & Rigau, 2015). Likewise, it is considered a technology in development, which is used to design new personalized food products with complex geometries, and adapted textures and allows to control of the nutritional value of the processed product (Reiss et al., 2021).
The term "3D bioprinting" was coined in 2004 by Hsieh et al., who used a prototype bioprinting system capable of printing 12 unique biological fluids, in parallel, to create arrays. This system used a rack of 12 stainless steel ejectors operated by a non-contact piezoelectric drive. Due to the high frequency of droplet ejection, they were able to achieve a true print-on-the-fly mechanism. One of their goals was to define a device that would provide precise control of microscale droplet delivery and efficient use of liquid, while also serving as a storage vessel for a unique biomaterial. Important parameters for their research design included being compatible with biological fluids, having minimal residual volume, being able to generate controllable and repeatable droplet volume, and taking advantage of simple and well-understood manufacturing processes (Hsieh et al., 2004).
It should be noted that in 2005, Philip Ball explained that the most common technique for creating artificial tissues involves growing cells on a polymer scaffold, which has been used for years to make artificial skin grafts from cultured fibroblasts. within a porous and biodegradable scaffold of a material such as collagen. However, three-dimensional tissues (artificial organs) are more challenging due to the problem of vascularization. Furthermore, without a blood supply, cells located deep within a scaffold matrix will quickly die. One option is to first grow the vasculature on a properly shaped tubular scaffold and then grow the other cells around it, a slow process at best. And it's difficult, with the scaffold approach, to reproduce complicated three-dimensional mixtures of cell types of the kind found in real organs. So bioprinting aims to address these issues, is considered an extension of rapid prototyping technology developed to create complex shapes in polymers and ceramics by building them as a series of two-dimensional slices. This inkjet technology is used to deposit material in the form of an "ink" that can be cured or sintered into a solid form. According to Philip Ball, the ink consists of groups of living cells that are printed on thin sheets of soft polymeric gel. This allowed them to add a fresh sheet of this gel "paper" for each successive layer, and the cells eventually adhered to form cohesive three-dimensional structures. Thomas Boland of Clemson University in South Carolina, for example, made tubes of contractile smooth muscle tissue from ring-shaped stacks of layers (Ball, 2005).
Currently, to design a 3D model, the first step is to have a 3D rendering software (CAD type), with which the model is subsequently saved in stereolithography (STL file). The printer software reinterprets the information in the STL file and transforms it into horizontal 2D sections that the printer will print additively to form the 3D food. 3D printing techniques that currently exist for the food industry include extrusion-based printing, selective laser sintering printing, folder jet printing, and inkjet printing (Pérez et al. 2020; Post, et al. al. 2020). However, extrusion printing has been the most used in the food industry, because it is applicable to a wide variety of food materials, maintaining their rheological properties (Sun, et al. 2015; Reiss et al., 2021). Likewise, this 3D bioprinting technology consists of 4 stages (Tan et al., 2018). Design of the structure (selection of cells and materials for the production of food ink); bioprinting process; post-bioprinting process (where a culture of the cellular structure is carried out), in which bioreactors can be used; and the evaluation of the structure, which serves to check the viability, structure, and functionality.
Extrusion-based printing, also known as fused deposition modeling, is based on precisely depositing the molten material using an extruder, which allows the continuous production of several filaments with a uniform cross-section and moving horizontally with respect to the printing platform. construction, along a prescribed path. This path is calculated by cutting software, which takes a 3D computer-aided design (CAD) model of a food structure. Virtually cuts it to form a series of horizontal cross-sections or layers, and uses algorithms to determine the path the nozzle should take to outline and fill each layer as the food material is extruded, taking into account user-specified fill patterns and fill density (Nijdam et al., 2022). There are three extrusion mechanisms in food 3D printing (Sun, et al. 2015), which are known as:
Screw-based extrusion: Food inks are placed in the sample feeder and transported to the tip of the nozzle by a movable screw.
Extrusion based on air pressure: Food materials are pushed into the nozzle by air pressure. This technique is useful for printing liquid or low viscosity materials.
Syringe-based extrusion: This technology consists of a cartridge containing the material to be printed, which ends in a nozzle or syringe with temperature control throughout the feeding system. The printing system is controlled by a motor and the material to be printed is deposited on a platform, which allows controlling the rheology of the material during the printing process. Extrusion systems can be a single or double nozzle or by means of a syringe, depending on the structural complexity of the food to be manufactured.
Likewise, in the food sector, there are materials that can be printable natively or also known as materials with inherent properties, which are components that can be adapted to the needs and development of various formulas. Among these materials are hydrogel, cake glaze, cheese, hummus, butter, and chocolates among others; powdered materials such as sugar and starch are also included here. On the other hand, there are traditional non-printable food materials, which have a nature that does not allow them to be printable, so hydrocolloids are added to be suitable for printing, among these hydrocolloids are carrageenan, gum arabic, xanthan gum and gelatin. Non-printable foods include fruits, meat, vegetables, and rice. In addition, there is also the possibility of being able to use alternative ingredients, among which fungi, seaweed, lupins, and insects are considered, to be a rich source of proteins, dietary fiber, and bioactive (Almeida-Bodero et al., 2019).
Among the ingredients that are currently indispensable in meat processing, NaCl plays an active role in 3D printing food. A particular case would be in the effect that NaCl has (in different concentrations) in the 3D bioprinting of surimi, allowing to improve the rheology of this product and control with greater precision the shape of this. (Wang et al., 2018; Yang et al., 2021). In addition, Yang et al. (2021) have also shown that NaCl and guar gum (polysaccharide obtained from the seeds of Cyamopsis tetragonoloba) allow obtaining a food ink suitable for the extrusion and elaboration of various meat products. It should be noted that in the elaboration of meat products by 3D bioprinting, it is necessary to measure the cooking performance, the characteristics of the texture, the state and distribution of moisture, and the microstructure of the product (Yang et al., 2021; Portanguen et al., 2019). In addition, the correct operation of the 3D printer must be considered, which is why the RSM-GA method is used in the process of making bioprinted chicken meat, which serves to simulate the effect of complex factors (nozzle diameter, filament diameter, nozzle temperature, and NaCl addition) on the formation accuracy of 3D printing.
On the other hand, there is also 4D printing, which is used to further modify the appearance of printed food. Over time, 3D printed food materials can undergo color, shape, or flavor transformations (i.e., the fourth dimension) induced by internal or external stimuli such as pH, water availability (e.g., hydration or dehydration), and heat (e.g., microwave cooking). As reviewed by Teng et al. (2021), functional ingredients such as colorants, dielectric regulators (ie, salts and syrup), flavors, and bioactive compounds are formulated in the base inks for 4D food printing. For example, color change of printed foods was achieved by double extrusion layers of two types of potato starch gels containing anthocyanin (as functional material) or lemon juice (as pH stimulus) (Teng et al., 2021; Ma & Zhang, 2022).
3. Bioethics and bioprinting
One of the first issues related to the bioprinting of food of animal origin is the feasibility of developing these technologies and the fulfillment of their moral validity of them, so this is a limitation in their commercial applications worldwide.
It should be noted that the main reason why the use of food bioprinting can be justified is the utilitarian philosophy, based on the need to save expenses in the excessive breeding of animals, speed up the obtaining of meat, progressively reduce a part of the pollution in the world, generate a quality product for the consumer and present a precise nutritional control. On the other hand, there is also the principle of misuse, which considers the nature of the moral fault of the producer, ignoring the biosecurity protocols required in the facilities to bioprint these products, the possible adverse effects, in which the proportionality between the good and bad effects is also evaluated, the social and religious aspects (Kai, 2020).
In the case of the U.S. Food and Drug Administration (FDA), there is a regulation of the laws governing the use of these products made by 3D bioprinters and how these relate to medicine and medical research, so this current framework does not allow to mitigate the possible risks for consumers of the product that is bioprinted, as well as not preventing certain requirements from being demanded from suppliers and manufacturers of the product, with the aim of studying this synthetic food from a bioethical perspective (Elemoso et al., 2020).
In the same way, it is necessary to evaluate and take into account the economic changes that can be triggered as a result of this new industry based on food bioprinting, since it could directly affect the meat sector, as is the case in countries with economies dependent on this source of income. On the other hand, the appearance of this new technology is accompanied by an ethic of sustainability, which has been presented as a potential alternative to replace traditional meat, the latter being considered an unsustainable variable for the environment.
Another moral perspective from the point of view of vegetarians and vegans is animal welfare, which is gaining more and more strength and makes meat consumption seen as an act of immorality. These ethical and moral issues drastically influence the current consumer and their food choices, so an alternative to mitigate this problem would be found in the use of biopsies in animals, which is a minimally invasive alternative and does not generate such great stress for animals. However, the goal of cultured meat is not only to meet the needs of these two views; but also, to satisfy the population that enjoys meat consumption and ensures its possible large-scale production in the future (Stephens et al., 2019).
Currently, the printability of salmon skin gelatin gels (SGGs) has been evaluated by studying their physical properties (rheological, textural, and printability), conclud ing that they could form stable structures after printing (Carvajal-Mena et al., 2022). This provides the basis for the study of 3D bioprinting using marine-derived gels, which could be used for dietary supplementation in humans.
On the other hand, various investigations have determined that the morphological information of the apple can be obtained through microtomography, which allows 3D printing of food, managing to reproduce the most outstanding characteristics of the microstructure of the apple tissue, in order to create snacks. cereal-based innovators (Derossi et al., 2022).
4. Utilities in industry and their applications in food production
In the medical sector industry, the 3D printer offers a large number of utilities in 3D models since they can be used for educational purposes, both for patients and students, doctors in training, and surgeons since they can play an important role in the process of reconstruction of complex surgical cases. It also opens up new opportunities for scientific research activities and can help elucidate physiological processes that are not yet fully understood. Another of the most outstanding utilities is the printing of 3D models of personalized implants, as is the case of the knee and hip prostheses made to "measure" (Ramadan & Zourob, 2021).
In the industry of art, collection, and education, 3D printers are used to make small objects, such as camera support, not so expensive pieces, among other materials of interest, which is achieved by using various materials, such as plastic or glass, even going so far as to propose the construction of houses (Sun et al., 2020). In the food industries, the 3D printer offers the opportunity to design new customized food products with complex shapes, geometries, textures, and nutritional value adapted to the demand of various consumers, it also allows the possibility of improving sustainability by reducing food waste or using alternative sources of nutrients (Li et al. 2021; Kim et al., 2022).
3D bioprinting extends to the food sector from the development of ingredients and products with functional effects, as well as to the manufacture of meat in vitro, being considered a promising technique, due to its advantages of scalability and controllability of the structure and composition (Handral et al., 2020; Kang et al. 2021). Likewise, this technology has the ability to achieve the spatio-temporal manipulation of several cells, which generates that bioprinting is one of the systems that best recreates the cellular microenvironment of tissues. And with it, cellular behavior at the laboratory scale (Tan et al., 2018). It should be noted that these machines only use the necessary material in the form of liquid, powder, or filaments that are then melted or solidified to create the final shape. Likewise, this technology has captured the attention of the commercial sector during the last decade, due to the potential it has to generate low-cost foods with a nutritional value similar to conventional foods. In recent years, research related to 3D printing of food is gaining momentum with increased attention from the academic field and its technical publications have also increased significantly (Kai, 2020). Among the most widely used bioprinters in food, the sector is those that work from a molten deposition (FDM) or thermoplastic extrusion.
The 3D printer has a nozzle that can move very precisely in three axes, through which it pours molten material in the form of threads that solidify immediately after leaving it. Among the foods that can be made would be meat, dairy products, cookies made from food ink made from insects, etc. (Almeida-Bodero et al., 2019). On the other hand, there are companies that generate products that resemble in texture, flavor, and nutritional value of meat products (Table 1).
There are currently several options for cell lines that allow the cultivation of meat, but refined research is needed to accurately determine the properties of cell lines that directly influence the process of making food bioinks (Figure 1). The best candidates are common types of stem cells, such as muscle stem cells, satellite cells, adipogenic stem cells, and induced pluripotent stem cells (Balasubramanian et al., 2021).
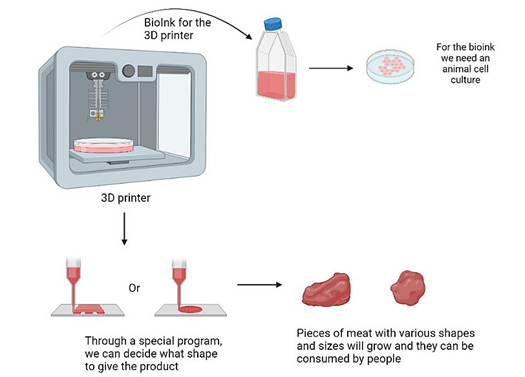
Figure 1 3D Bioprinting of meat from muscle stem cell bioinks of bovine origin. Adapted from Balasubramanian et al. (2021) & Derossi et al. (2021).
Table 1 Meat companies that use 3D bioprinters
Bioprinter Company | Species of cellular origin | Reference |
Meal Source Technologies, Aleph Farms, Fork and Goode, Meatech | Mammals | Farms (2021) |
BlueNalu | Seafood | Elemoso et al. (2020) |
Meat technologies of the future | Multiple species | Elemoso et al. (2020) |
Since bioinks transport cells and support the structure of the bioprinted food, they must possess good mechanical properties and biocompatibility, which are key challenges in 3D bioprinting. Hydrogels are currently the most widely used bioinks on the market, due to their high-water content, physical structure, mechanical properties, and 3D network structure. They also mimic the extracellular matrix around cells, provide a favorable environment for cell growth, and enable intercellular interactions and the exchange of biochemical signals (Chimene et al., 2019; Kiyotake et al., 2019; Li et al., 2021; Kamalapuram et al., 2021).
It is worth mentioning that this technology has also allowed better access to attractive and nutritious food. That is why in some hospitals or homes for the elderly, a food product made specifically for people who have dysphagia or problems swallowing food is being developed; additionally, it has been determined that homogeneous foods (such as pures) are relatively unappetizing, which leads to a deficient oral intake of both food and liquids. For this reason, this food production technique has allowed the transformation of shapeless porridge into 3D structures, causing patients to recognize the product as conventional food (Tan et al., 2018). In addition, the specific nutritional content of these foods can be customized, which is achieved by carefully adapting the formula of the food ink. For example, it has been possible to make 3D prints of a concentrated orange gel enriched with vitamin D (Azam et al., 2018).
In 2019, the Israeli company Aleph farms developed a method for producing cultured meat fillets. This method mimics the natural process of muscle regeneration that occurs within the cow's body but under controlled conditions. Aleph Farms has demonstrated its ability to grow different types of cells, which after 3 to 4 weeks, allows for forming a fillet of beef in thin slices with a 3D structure (Farms, 2021).
4.1. Food production for astronauts
In space, food is one of the biggest drawbacks that the human species can have in the face of the development objectives of long-duration missions. Currently, food consumed by astronauts aboard the International Space Station (ISS) is pre-packaged from Earth. There are prototypes of cultivation, such as the Veggie system, which provides fresh food and uses low resources. However, the production of food grown in the Veggie system is not viable for a long-term mission, due to the time it takes for the growth of the crop and the nutritional and liquid requirements that are needed (Stutte et al., 2011; Ates & Zhang, 2007).
For this reason, the bioprinting alternative emerges as a technological necessity for future space travel, such as trips to the Moon and Mars in the following 5 and 10 years, respectively. Bioprinting will help reduce the gaps given by the food need in space because it will be possible to produce food of high nutritional quality (in particular protein foods) satisfying the dietary needs of astronauts as many times as necessary (Axpe et al., 2020).
There are companies that have been involved in the bioprinting of food for astronauts, among these is Aleph farms, which managed to print meat in 3D on the International Space Station. Its partner in this project is the company 3D Bioprinting Solutions, which has lent its technology to facilitate this advance. In addition, the Russian Space Agency (Roscosmos) is also involved in this project. Aleph Farms' mission is to provide safe and nutritious meat anytime, anywhere on Earth, while utilizing minimal resources (Farms, 2021).
Another space agency that has been experimenting with bioprinting in space for years is the National Aeronautics and Space Administration (NASA). NASA has funded the systems and Material Research Corporation's advances with the goal of producing food for astronauts using 3D bioprinting. In this way, edible structures with the proper texture of protein, starch, and fat needed in the astronauts' diet would be created (Johnson, 2019).
5. Advantages and disadvantages of bioprinting
3D bioprinting brings with it a series of ethical, economic, and technological challenges, which can be more notorious or less problematic depending on the destination or function of bioprinting, in this context, the main advantages of allocating bioprinting to the food area as in meat printing, would cause one of the main disadvantages of bioprinting of animal tissue that exists in medicine to no longer be taken into account, this is the problem of vascularization since it would not represent an inconvenience if the meat is intended for food. Another advantage will be the precision and saving of materials that printing offers. According to Berman (2012), this technique minimizes the waste of inputs, allowing to obtain results efficiently, quickly, and accurately.
Among the main disadvantages of allocating this technique to the food industry, we would have in the first place a clear economic and technological disadvantage, depending on the technological scope and economic level of the company or private laboratory, added to the knowledge in software that is required to make it work correctly with the precise materials that the bioprinter will require to perform this work. According to Escudero (2018), inadequate control and precision of the factors that will keep the rheological properties of the food that will be printed optimally will be affected in terms of its quality and subsequent storage. According to these characteristics, another disadvantage would be not knowing what type of materials to use for the elaboration of the "pasta" that will be used for the elaboration of the food, which must be chosen precisely so that it can be preserved for long periods of time, if you want to give it a food use, possibly focused on the space mission food industry.
6. Critical appraisal
This work deals with 3D bioprinting of food as a technology in development that has allowed in recent years to design new personalized food products, which generates faster production, use of inputs and reduction of waste, food preparation with complex geometries, as well as control over the texture and nutritional value of the processed product. Likewise, the most widely used 3D bioprinting technique in the food industry is extrusion, because it is applicable to a wide variety of food materials, maintaining their rheological properties. In addition, in various research projects, it has been proven that this food production technique will reduce the emission of greenhouse gases (which are generated largely by the excessive number of livestock in the world), reduce invasive methods of obtaining meat, and solve the problem of food shortages due to global population growth. One of the projects that have had the greatest relevance in the world has been at NASA, with the application of 3D bioprinters for the preparation of food in space travel.
Currently, in the latest advances in this technology, 4D bioprinting has been achieved, which allows the appearance of printed food to be modified with greater precision, whether at the level of color, various types of shapes, or flavor, caused by stimuli. internal or external such as the pH, the amount of water, and the cooking heat of the food.
However, despite all these technological advances, it is still necessary to carry out further research on the equipment, supplies, and software of equipment, in order to guarantee that the products that are produced today and in the future are of high quality.
Interest in this technology has been increasing year after year (Figure 2), with optimization (2018) and optimization (2019) studies appearing.
Table 2 Advantages and disadvantages of 3D food bioprinting
Advantages | Disadvantages |
There is no vascularization in all bioprinted meat products, which simplifies the production process at an industrial level. Allows greater precision and material savings, minimizing the waste of supplies. Allows you to customize the nutritional content of these foods. | Each bioprinted food requires specific software to work properly. If there is no proper control of the rheological properties of the food to be printed, it will be affected in terms of its quality and subsequent storage. This technique would have an economic disadvantage, due to the cost of the equipment (as is the case of a 3D bioprinter, bioreactors, etc.) |
Progressively reduce part of the pollution, greenhouse gas emissions generated by excessive animals. It is an alternative technology that emerges as a necessity for future space travel. | The production of bioprinted food at an industrial level requires going through an ethics committee, which evaluates whether it complies with the standards established in the country and the ethical and moral aspects. |
Adapted from Berman (2012); Azam et al. (2018); Kirillova et al. (2020); Axpe et al. (2020); Escudero (2018); Mateus-Malagón & Paredes-Acosta (2020); Kai (2020); Elemoso et al. (2020).
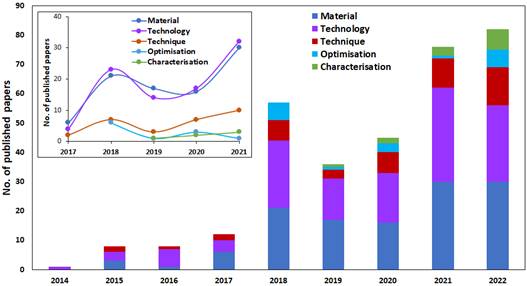
Figure 2 Detailed breakdown of the number of publications for each 3D food printing classification (TITLE-ABS-KEY: “3D food printing” + Search criteria [Material, Technology, Technique, Optimisation, Characterisation] in SCOPUS from the publication year 2014 to 2022; inset: 5 years publication trend from the year 2017 to 2021.
7. Conclusions
The biggest advantage of bioprinting in food is the speed of production compared to traditional manufacturing methods. This technique generates complex shapes and parts of various foods used in the common diet, also controlling the texture and nutritional value of it. In addition, this makes it possible to reduce environmental pollution and take advantage of production materials. These products are presented as an irrefutable solution to the problem of feeding in places of scarce resources such as space and areas not suitable for animal husbandry. Future studies should focus on optimizing processes, as well as looking for new applications.