1. Introduction
Tomato (Solanum esculentum L.) is one of the most important plant species of agricultural importance worldwide. Biotic factors such as diseases, especially root-knot nematodes (Meloidogyne spp.) cause severe damage and losses in different crops, mainly due to wide geographical distribution and their potential to infect a wide variety of plant species (Calderón et al., 2022). In Ecuador, the genus Meloidogyne is an important pathogen that can affect Solanaceae (S. lycopersicum, S. betaceum, S. quitoense and Capsicum annuum L.), as well as Musaceae (Musa sp.), rice (Oryza sativa L.), common bean (Phaseolus vulgaris L.), and lettuce (Lactuca sativa L.) (Bridge, 1976; Erazo-Sandoval et al., 2020; Calderón et al., 2022).
Among the most important and most studied Meloidogyne species are M. arenaria, M. javanica, and M. incognita (dos Santos et al., 2012; Calderón et al., 2022; Wen et al., 2022). This latter species causes mainly root damage (galls), and other aerial parts symptoms i.e., stunting, wilting, or chlorosis (yellowing) (Sikora et al., 2008; Calderón et al., 2022). As usual, infected plants root system is stunted leading to poorly growing crops (Muimba-Kankolongo, 2018). Symptoms are particularly stronger and severe when plants are infected soon after planting, being able to reduce crop productivity and cause between 68% and 100% fruit production damage due to product’s low quality (Hussey & Barker, 1973). M. incognita is a sedentary endoparasitic nematode that occurs in more than 2000 plant species (Jung and Wyss, 1999), which makes it difficult to control. A total of five Meloidogyne species have been identified in Ecuador, highlighting M. incognita as the most frequent, followed by M. arenaria, M. graminicola, M. hapla and, M. javanica (Calderón et al., 2022).
Root-knot nematode management is generally done by using nematicides. However, the prohibited use of methyl bromide, 1,3-dichloropropene and cadusafos, the main used pesticides, let the currently management of this pathogen with ineffective options (Sikora et al., 2008). Thus, the use of rhizobacteria is shown as an efficient strategy for phytopathogenic nematodes management (Hallmann et al., 1998; 1999; Sikora et al., 2008; Chávez-Arteaga et al., 2018). Agricultural soils have a high microbial diversity associated with different plant species root systems, highlighting the presence of Plant Growth Promoting Rhizobacteria (PGPR) (Cedeño-Moreira et al., 2020). These microorganisms can help in nitrogen fixation, increase the availability of nutrients in the rhizosphere, production of antibiotics, phytohormones, and siderophores (Siddiqui, 2005), as well as production of biofilms in different plant species (Haque et al., 2020). Nevertheless, secondary metabolites produced by PGPR in the plant rhizosphere also may cause direct negative effects on nematodes, for example, nematode eggs membrane rupture, and drastically reducing pathogen populations in underground tissue (El-Sherif et al., 1999, Siddiqui et al., 2005; Montes et al., 2016).
The diversity and number of existing PGPR are varied, as well as their use in different pathosystems. In recent years, rhizobacteria like Pseudomonas protegens (CHA0 strain) and Pseudomonas veronii (R4 strain) have shown a negative effect against nematodes (Canchignia-Martínez et al., 2017; Montes et al., 2016). Both microorganisms produce the auxin indole-3-acetic acid (IAA), which can help develop the grape and soybean root system (Peñafiel-Jaramillo et al., 2016; Cedeño-Saavedra et al., 2017). Recently, seven rhizobacteria obtained from banana landraces rhizosphere in Los Ríos, Cotopaxi, and Bolívar provinces, Ecuador, and used in the present work, could be considered PGRP-like candidates due to their antagonistic activity (Chávez-Arteaga et al., 2018). Two of these strains, Acinetobacter calcoaceticus (BM2-12 strain) and Serratia marcescens (PM3-8 strain), showed negative effect in vitro and in vivo against Phytophthora palmivora, the causal agent of the black pod, as well as capacity plant growth-promoter on cocoa seedlings (Cedeño-Moreira et al., 2020).
Studies on the use of PGPR in different crops of agricultural importance are very scarce. Thus, the present work aimed to evaluate the in vitro and in vivo antagonist activity of rhizobacteria against M. incognita and quantify the plant-growth promotion capacity in tomato plants infected by the nematode.
2. Materials and methods
Biological material
Tomato plants of cv. Floradade were used in all experiments. Meloidogyne incognita root-knot nematode (local strain Uteq-Mi1) used here, was identified using morphological (Taylor & Sasser, 1983) and molecular techniques (polymerase chain reaction - PCR according to Chávez-Arteaga et al., 2018). For selective amplification of target nucleotide sequences were chosen two primer pairs: Finc (5´CTCTGCCCAATGAGCTGTCC3´) and Rinc (5´CTCTGCCCTCACATTAAG3´) (Hesar et al., 2022), and Inc-K14F (5´CCCGCTACACCCTCAACTTC3´) and Inc-K14R (5´GGGATGTGTAATGCTCCTG3´) (Wen et al., 2022). See Figure A1 (Appendix) for more details. The plant growth-promoting rhizobacteria (PGPR) shown in Table 1 were employed.
Table 1 Plant growth-promoting rhizobacteria used in the present research, and that produce (+) or not the secondary metabolites Protease (PR), hydrogen cyanide (HCN), pyrrolnitrine (Prn), diacetylfloroglucinol (2,4-DAPG), siderophores (Sd) and indole-3-acetic acid (IAA)
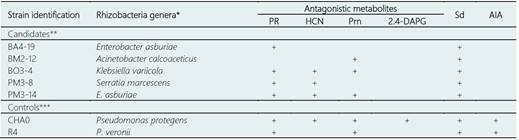
* Identity of 16S rRNA gene to information from GenBank NCBI.
** Chávez-Arteaga et al. (2018).
*** Canchignia-Martínez et al. (2017); Montes et al. (2016); Peñafiel-Jaramillo et al. (2016); Cedeño-Saavedra et al. (2017).
In vitro experiments
Assessment of nematode egg hatching
Egg masses of M. incognita were obtained of tomato roots with root-knot galls symptoms. These tissues were washed with sterile distilled water. Egg mass was collected with a sterilized tooth stick (65x2 mm) and placed on a sterile glass slide containing sterile distilled water and 50 µL of chloramphenicol.
PGPR (100 µL at a concentration 1 × 108 cfu mL-1) was placed on Petri dishes containing King B liquid medium. Subsequently, egg mass (±100 eggs) was placed on these plates. The microorganisms were incubated at 27 ± 2 °C and 12-hour photoperiod for 6 days. On this day, the number of empty eggs in an area of 0.5 cm2, an average of 50 eggs was evaluated using an optical stereo microscope at 5x magnification (model BX40, Olympus, Japan) coupled to a photographic camera with ISCapture Professional Imaging software.
Determination of nematodes mortality (J2 stage)
Like the methodology used in the previous variable, M. incognita egg masses were obtained from tomato roots with root-knot galls symptoms. These masses were placed into PCR Eppendorf tubes containing 50 μL of sterile water. Four days later, 5 μL of this suspension containing an average of 20 juvenile nematodes in J2 stage, were placed on Petri dishes containing King's B agar media and PGPR. At 8, 16 and 24 h after, nematodes were recovered and re-suspended in sterile water to verify their mobility or death.
Experiments in greenhouse conditions
Plant growth conditions
Seeds were sown in 12-cell expanded polystyrene (EPS) foam (Styrofoam) trays containing 46 cm3 of a sterilized mixture (121 °C for 20 min) of peat and clay loam soil (1:1, Novarbo seedling, black C1B). Cells were irrigated according to the crop needs. Seedlings were kept under greenhouse conditions (25 ± 4 °C and 12 h of light).
Inoculation of plant growth-promoting rhizobacteria
PGPR were grown on King's B agar media in dishes and maintained at 25 °C (±1 °C) for 48 h. The bacterial culture (100 mL of a concentration 1 × 108 cfu mL-1) was centrifuged (3000 g x 15 min), washed twice with sterile phosphate-buffered saline (pH 6.5), and finally re-suspended in the same solution for its inoculation.
Seedlings (19 days after sowing) were carefully taken out of the substrate, their roots thoroughly washed with tap water, surface disinfected in 0.1% (v/v) NaOCl solution for 1 min and rinsed twice with sterile deionized water. Then, plants were inoculated by rhizobacteria suspension root dipping for 10 min. Plants whose roots were dipped in distilled water served as control. After inoculation, seedlings were immediately planted in 180 cm3 pots containing an infested mixture nematode of organic compost and vermiculite (1:1) and kept under the same conditions described before. The substrate was kept moist and irrigated twice every week with 1.7 mL nutrient solution per g of substrate. Subsequently, two applications were made at 4-day intervals with each of the strains (10 mL of a concentration 1 × 108 cfu mL-1), placing the suspension at 5 cm of crow-root.
Nematode inoculum
M. incognita eggs and juveniles were obtained from tomato roots of cv. Floradade. These structures were extracted with NaOCl through the centrifugal flotation method (Hussey & Barker, 1973), using a shaking incubator (Incu-Shaker™ Shaking Incubators, Benchmark Scientific, U.S.A). Plants of this same cultivar were inoculated with 4000 nematodes (eggs and juveniles), grown for two months on the same sterilized substrate, and kept under the same conditions described before.
Seedlings at the first true leaf stage (15 days after inoculation) were planted in 180 cm3 pots containing sterilized substrate. One week after transplantation, plants were each inoculated with a suspension calibrated for 1000 eggs and J2 per mL of M. incognita, in holes in the soil around the stem of each plant. The plants were kept in a greenhouse at 25±4 °C, 12 h of light and watered as needed.
Quantification of plant height and root weight
On the 30th day after transplanting, plant height was measured (distance between the ground surfaces to the point of growth of each plant) using a graduated ruler. After this, the tomato root system was carefully removed and washed with tap water, dried on a paper towel, and weighed (g) on a precision balance (Kern 440, China).
Assessment of root-knot symptoms in aerial and underground tissues
At 30 days, the effect of rhizobacteria in infected tomato plants with root-knot nematode M. incognita was assessed. Symptoms i.e., root galls, dwarfism and leaf chlorosis were assessed qualitatively. Then, plants were collected, and their roots carefully removed. In these tissues, the gall numbers induced by nematodes were quantified. Immediately, root galls were liquefied and passed through sieve with a mesh size of 500, 90, and 25 μm. Eggs and J2 nematodes recovered in the 25 μm sieves were observed, counted, and expressed per fresh weight (g) of tissues, using an optical stereo microscope at 10x magnification (Olympus, Japan).
Experimental design and statistical analysis
The experiments were carried out in a completely randomized design with eight treatments and three replications (each one containing two plants).
After verification of homogeneity of the variances (Bartlett’s test) of the data sets, these were subjected to analysis of variance. Student's t-test (p ≤ 0.05) was used to compare treatments among the times evaluated of the variable Plant height, while Scott Knott's test (p ≤ 0.05) was used for the grouping of means of each variable. Also, Pearson's analysis was performed to investigate the correlation among all variables evaluated. Statistical analyzes were performed using the software Prism Windows 5.04.
3. Results and discussion
Although root-knot nematode (M. incognita) is a major disease of tomato crops in Ecuador, little attention has been given to its identification, and even worse to its management using environmentally friendly technology. In our study, we compared the response of tomato plants infected by M. incognita strain Uteq-Mi1, to the application or not with strains of seven rhizo-bacteria, some of these strains being Ecuadorian, evaluated both in in vitro and greenhouse conditions.
In vitro experiments
Nematode egg hatching
A higher number of nematode hatched eggs (p < 0.01) was observed in control dishes, at 4 (91 eggs) and 6 (98 eggs) days when compared to those treated with PGPR (4 days: 10 eggs; 6 days: 12 eggs, in average). Application of CHA0 (0.7 eggs in average), PM3-8 (2.8 eggs in average), R4 (3.0 eggs in average), and PM3-14 (6.8 eggs in average) strains significantly re duced the nematode egg hatching, in both times analyzed (Figure 1).
Nematode mortality
The mortality of J2 nematodes was significantly increased when applied all rhizobacteria at three times analyzed. Nevertheless, the dishes containing CHA0 strain showed a nematode mortality of 74% in average, when compared to their control (6% in average) and the other treatments (38% in average), analyzed over time (Figure 2). Additionally, regardless of the application or not of each strain, the nematode mortality increased over time.
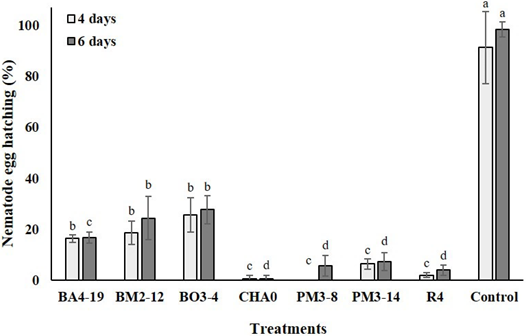
Figure 1 Number of nematode hatched egg (%) Meloidogyne incognita in Petri dishes containing Enterobacter asburiae (BA4-19), Acinetobacter calcoaceticus (BM2-12), Klebsiella variicola (BO3-4), Pseudomonas protegens (CHA0), Serratia marcescens (PM3-8), E. asburiae (PM3-14) and P. veronii (R4), or without rhizobacteria (only sterile distilled water), at 4 and 6 days.
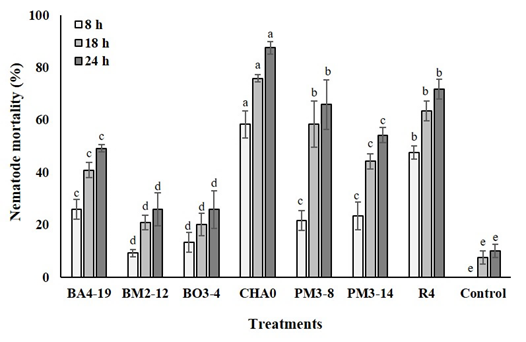
Figure 2 Mortality of Meloidogyne incognita nematodes J2 stage (%) in Petri dishes containing Enterobacter asburiae (BA4-19), Acinetobacter calcoaceticus (BM2-12), Klebsiella variicola (BO3-4), Pseudomonas protegens (CHA0), Serratia marcescens (PM3-8), E. asburiae (PM3-14) and P. veronii (R4), or without rhizobacteria (only sterile distilled water), at 8, 18 and 24 h.
All rhizobacteria significantly reduced and increased the nematode egg hatching and its mortality (J2 stage), respectively, showing even a relation between both variables in all analyzed times in in vitro conditions. Nevertheless, CHA0, R4, PM3-8, and PM3-14 strains were shown to be more effective in inhibiting the nematode egg hatching, while the first cited strain had the ability to kill a greater number of nematodes, in comparing to control and the other rhizobacteria, analyzed at over time. To our best knowledge, the effectiveness of CHA0 and R4 strains in killing nematodes has already been verified, but mostly in other crops. Indeed, CHA0 strain can inhibit egg hatching by 60%, as well as it can kill between 55 and 63% of juvenile J2 nematodes of M. javanica (Hamid et al., 2003; Siddiqui and Shaukat, 2003). On the other hand, R4 strain can affect the viability of Xiphinema index (Canchignia-Martínez et al., 2016; Montes et al., 2016). It is known that M. incognita is affected by strains of Serratia marcescens and Pseudomonas fluorescens in cotton (Hallmann et al., 1998; 1999). Therefore, this is the first report of CHA0, R4, PM3-8, and PM3-14 strains showing antagonistic activity against M. incognita isolated from tomato roots in in vitro conditions.
We attribute the antagonist effect against M. incognita shown by the strains, to the production of volatile compounds. It is known that rhizobacteria produce diacetylfloroglucinol (2,4-DAPG), hydro-gen cyanide (HCN), pyrrolnitrine (PrnR), iron-chelating siderophores (ICS), antibiotics, protease (PR), and indole-3-acetic acid (IAA) (Hamid et al., 2003; Siddiqui & Shaukat 2003; Canchignia-Martínez et al., 2016; Montes et al., 2016). These substances have been implicated in the reduction of deleterious effects and pathogenic rhizosphere microorganisms, creating an environment more fa vorable for the plants root growth (Siddiqui, 2005). For example, HCN inhibits the cytochrome oxidase of nematode cells, inhibiting its mitochondrial respiration causing paralysis and rapid death (Cunningham et al., 1997). This substance and 2,4-DAPG and PR can even function as nematicides, by degrading the cell wall of both eggs and J2 stage nematodes, finally causing lysis (Siddiqui and Shaukat, 2003; Timper et al., 2009). Therefore, it is plausible to assume that volatile bioactive compounds produced by the rhizobacteria understudy, can affect the nematode egg hatching and its mortality (J2 stage).
Our results showed that the nematode mortality increased over time, regardless of the application or not of each strain. If we observe the control treatment, we realize that the mortality also increased. In this way, we can infer that in addition to the nega tive effect induced by volatile bioactive compounds on the nematodes (Siddiqui & Shaukat 2003; Siddiqui, 2005), these tend to die over time.
Experiments in greenhouse conditions
Plant height and root weight
Both plant height (20 days: p < 0.05; 30 days: p < 0.01) and root weight (p < 0.01) varied among treatments (Figure 3). Tomato plants treated with BM2-12 and R4 strains obtained a higher height, at 20 (32 cm in average) and 30 (55 cm in average) days, in comparison to other treatments (Figure 3A). Additionally, an increase in height was observed in tomato plants’ time course, but not in plants treated with the BA4-19 strains and the untreated ones. Interestingly, only R4 strain generated a negative effect against nematodes, while the BM2-12 strain was less effective in the in vitro experiments. It is known that some rhizobacteria isolates like P. fluorescens produce phytohormones, generating greater plant growth (Siddiqui & Shaukat, 2003; Hashem & Abo-Elyousr, 2011). Perhaps the rhizosphere of tomato plants is being stimulated by some volatile compound present in both bacteria, resulting in greater height.
Tomato plants treated with CHA0, PM3-14 and R4 strains showed at lower root weight (values less than 3 g), compared to their control and the other treatments (4.2 g in average, Figure 3B). Although this result is contrary to expectations, Pearson's analysis shows a significant correlation among root weight with gall and nematode numbers (Table 2). Therefore, the number of galls and J2 stage nematodes influenced the weight of underground tissues. On the other hand, the null correlation between plant height and the other variables (Table 2) shows a differentiated response by tomato plants to each of the rhizobacteria, and different metabolic pathways can be activated. However, more studies are needed to understand these processes.
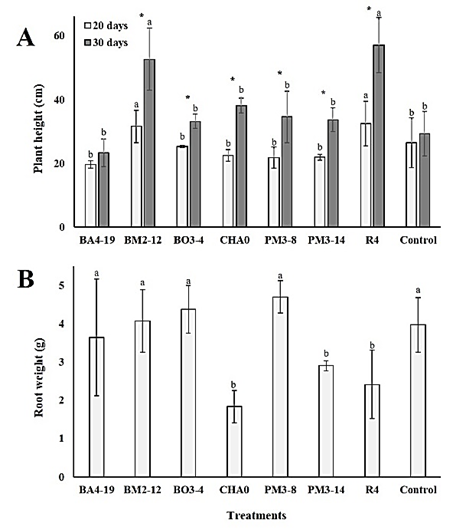
Figure 3 Plant height (cm, A) and root weight (g, B) in tomato plants infected by Meloidogyne incognita and treated or not with Enterobacter asburiae (BA4-19), Acinetobacter calcoaceticus (BM2-12), Klebsiella variicola (BO3-4), Pseudomonas protegens (CHA0), Serratia marcescens (PM3-8), E. asburiae (PM3-14) and P. veronii (R4). Plants whose roots were dipped in distilled water served as control.
Assessment of root-knot symptoms in aerial and underground tissues
Independent of the application or not of rhizobacteria, all tomato plants showed symptoms caused by root-knot nematode M. incognita, at 30 days after inoculation (Figure 4). However, these symptoms (root galls, dwarfism, and leaf chlorosis) were stronger and intense in infected plants without rhizobacteria (Figure 4 C and F), in comparison with their respective plants treated with Bm2-12 (Figure 4 A and D), CHA0 (Figure 4 B and E) and the rest of strains. All symptoms have been observed in tomato plants and other plant species in Ecuador (Bridge 1976; Erazo-Sandoval et al., 2020; Calderón et al., 2022), leading to poorly growing and low fruit production in crops (Muimba-Kankolongo, 2018).
Roots of untreated plants with any of the strains showed a higher number of galls (Figure 5A) and nematodes (J2 stage) (Figure 5B), when compared to those treated (p < 0.01). Practically, the behavior was similar in both variables. A lower number of galls (Figure 5A) were observed in plants treated with BM2-12 (318 galls), CHA0 (59 galls) and R4 (300 galls) strains, compared to those control (3099 galls) or the rest of the treatments (1341 galls on av erage). On the other hand, the application of BM2-12 (233 nematodes g-1 fresh weight) and CHA0 (173 nematodes g-1 fresh weight) strains (Figure 5B), significantly reduced the number of nematodes in the roots of tomato plants, compared to those control (1467 nematodes) or with the rest of treatments (771 nematodes g-1 fresh weight on average).
Lower galls and nematode numbers were observed in roots of tomato plants treated with BM2-12 and CHA0 strains. However, the R4 strain also induced a reduction of gall numbers in underground tissues, compared to those in control or with the rest of the strains. BM2-12, CHA0, and R4 strains produce the enzymes protease and catalase, as well as HCN and PLT (Siddiqui & Shaukat 2003; Canchignia-Martínez et al., 2016; Montes et al., 2016). It is also known that some strains of P. fluorescens can reduce galling by M. incognita and their population in roots (Siddiqui et al., 2005; Hashem & Abo-Elyousr, 2011). Although many strains used in the present experiments showed a negative effect against the nematode M. incognita, the metabolite compounds role in tomato plants remains poorly understood (El-Sherif et al., 1999). Hence, elucidating the effect of these substances on the pathogen, as well as in tomato plants will be an exciting challenge for future research.
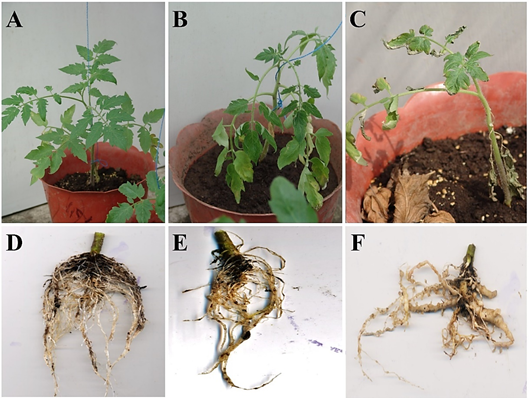
Figure 4 Symptoms in tomato plants infected by root-knot nematode Meloidogyne incognita and treated or not with rhizobacteria strains, at 30 days. A and D, slight leaf wilting and low growth of galls in root of plants infected and treated with Acinetobacter calcoaceticus strain Bm2-12. B and E, slight leaf wilting, epinasty, chlorosis and root galls in plants infected and treated with Pseudomonas protegens strain CHA0. C and F, dwarfism, chlorosis and root galls stronger and intense in plants only infected with nematodes.
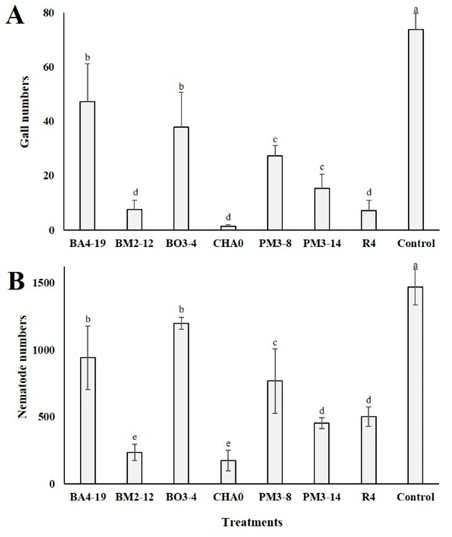
Figure 5 Gall and nematode numbers in roots of tomato plants infected by Meloidogyne incognita tomato plants treated or not with Enterobacter asburiae (BA4-19), Acinetobacter calcoaceticus (BM2-12), Klebsiella variicola (BO3-4), Pseudomonas protegens (CHA0), Serratia marcescens (PM3-8), E. asburiae (PM3-14) and P. veronii (R4). Plants whose roots were dipped in distilled water served as control.
Pearson's correlations among variables
Results of Pearson's correlation analysis among all variables can be observed in Table 2. Nematode egg hatching at 4 and 6 days was correlated with nematode mortality at 8, 18 and 24 days (p-values among 0.0005 and < 0.0001). The variables plant height at 20 and 30 days, only correlated with each other (< 0.0001). The nematode numbers at 8, 18 and 24 h were correlated with each other (< 0.0001), but also with root weight (p-values among 0.0003 and 0.0077), as well as with gall (p-values among 0.0006 and 0.0007) and nematode (p-values among 0.0008 and 0.0380) numbers. The gall numbers in root tissues were correlated with nematode egg hatching at 4 and 6 days (< 0.0001), as well as with plant height at 30 days (0.0030) and nematode J2 numbers (0.0399). Finally, root weight was correlated with gall (0.0081) and nematode stage J2 numbers (< 0.0001). These responses observed in M. incognita caused by rhizobacteria in both conditions show the importance of Ecuadorian strains with antagonistic capacity against this little-studied pathogen. However, more studies are needed to understand the pathogen-rhizobacteria-plant interaction and the effect of strains on tomato fruit production.
In this work, we report for the first time the ability of S. marcescens (PM3-8 strain) and E. asburiae (PM3-14 strain) to inhibit egg hatching, and to kill a greater number of nematodes (J2 stage), compa-ring herself with the strains CHA0 and P4. Also, we verified that the roots of tomato plants treated with A. calcoaceticus (BM2-12 strain) showed a higher height, as well as a fewer number of galls and nematodes in underground tissues.
Table 2 Pearson's correlation among nematode egg hatching at 4 (NEH4) and 6 (NEH6) days, nematode mortality at 8 (NM8), 18 (NM18) and 24 (NM24) hours, plant height at 20 (PH20) and 30 (PH30) days, root weight (RW), and gall (GN) and nematode numbers (NM), variables evaluated in laboratory or greenhouse conditions. The values shown for each Pearson's correlation are the coefficient (r) and the value resulting from regression analysis (p-values between 0.0001 and 0.05). Cells with significant correlations were highlighted with gray-colored
NEH4 | NEH6 | NM8 | NM18 | NM24 | PH20 | PH30 | RW | GN | NM | |
NEH4 | - | 0,9771 | 0,4284 | 0,5696 | 0,5850 | 0,0040 | 0,1034 | 0,0631 | 0,6424 | 0,0273 |
- | <0,0001 | 0,0005 | <0,0001 | <0,0001 | 0,7698 | 0,1254 | 0,2365 | <0,0001 | 0,4398 | |
NEH6 | - | 0,4640 | 0,5851 | 0,6088 | 0,0162 | 0,0802 | 0,0940 | 0,6256 | 0,0509 | |
- | 0,0002 | <0,0001 | <0,0001 | 0,5529 | 0,1801 | 0,1452 | <0,0001 | 0,2893 | ||
NM8 | - | 0,8084 | 0,8056 | 0,0058 | 0,0649 | 0,4630 | 0,4042 | 0,4061 | ||
- | <0,0001 | <0,0001 | 0,7241 | 0,2298 | 0,0003 | 0,0008 | 0,0008 | |||
NM18 | - | 0,9859 | 0,0175 | 0,0556 | 0,2891 | 0,4188 | 0,1813 | |||
- | <0,0001 | 0,5380 | 0,2675 | 0,0067 | 0,0006 | 0,0380 | ||||
NM24 | - | 0,0185 | 0,0583 | 0,2814 | 0,4110 | 0,1843 | ||||
- | 0,5264 | 0,2555 | 0,0077 | 0,0007 | 0,0363 | |||||
PH20 | - | 0,6794 | 0,0201 | 0,0300 | 0,0003 | |||||
- | <0,0001 | 0,5092 | 0,4185 | 0,9310 | ||||||
PH30 | - | 0,0101 | 0,3362 | 0,0264 | ||||||
- | 0,6410 | 0,0030 | 0,4478 | |||||||
RW | - | 0,2782 | 0,8666 | |||||||
- | 0,0081 | <0,0001 | ||||||||
GN | - | 0,1781 | ||||||||
- | 0,0399 | |||||||||
NM | - | |||||||||
- |
4. Conclusions
In laboratory conditions, all rhizobacteria negatively affected the nematodes, standing out P. protegens (CHA0), S. marcescens (PM3-8), E. asburiae (PM3-14), and P. veronii (R4) by reducing the nematode egg hatching, as well as CHA0 strain for being the most effective in killing nematodes of M. incognita. In greenhouse conditions, tomato plants treated with A. calcoaceticus (BM2-12) and R4 strains showed a higher height, and the plants treated with CHA0, PM3-14 and R4 strains showed a lower root weight. Independent of the application or not of rhizobacteria, all tomato plants showed symptoms caused by root-knot nematode, but these were stronger and more intense in infected plants without rhizobacteria. A lower number of galls was observed in plants treated with BM2-12, CHA0 and R4 strains. Also, the number of nematodes in roots was reduced with the application of BM2-12 and CHA0 strains. To the best of our knowledge, this is the first report on the capacity of S. marcescens and E. asburiae to plant growth-promoter and root-knot nematode controller, respectively, in tomato plants. Although many variables, both laboratory and greenhouse conditions, were significantly correlated in this research, some exceptions were observed, for example, the null correlation between plant height and the other variables. Even though further studies are needed to understand the pathogen-rhizobacteria-plant interaction and the effect of strains on tomato fruit production, our study shows practical results about the biological control of M. incognita in tomato crops.