1. Introduction
Soil salinity has negative effects on the morphology, physiology and biochemistry of plants. The increase in osmotic pressure in the soil decreases the absorption of water by the root system; excess sodium (Na+) and chloride (Cl-) ions are toxic to cells; and ionic imbalance affects the nutritional status of plants (Boari et al., 2019). In the world, more than 6% of the soil (800 million ha) in potential agricultural use is impacted by salinity, especially in arid and semi-arid regions (Afridi et al., 2019). In Peru, salinity affects 306,701 cultivable hectares on the coast (Ministerio de Agricultura y Riego [MINAGRI], 2015), especially in Piura, Trujillo and Lambayeque (Soca et al., 2016).
Salinity stress impacts plant growth reducing their yield (Afridi et al., 2019; Thiam et al., 2019). The cultivation of Solanum lycopersicum L. "tomato" is considered moderately sensitive to salinity (Boari et al., 2019). The critical level of soil salinity for tomato cultivation is 2.5 dS/m and it is sown in soils with up to 9.9 dS/m (Welle & Mauter, 2017); however, salinity drastically decreases water consumption, and affects plant height, biomass of stems and leaves, root density, fruit quality and weight negatively (Boari et al., 2019) and also decreases yield by 20-65% (Chen et al., 2016).
The cacti group succulent plants endemic to America such as Opuntia sp. “prickly pear” (Ministerio del Ambiente [MINAM], 2014) can develop in semi-arid and arid regions with prolonged drought, thanks to their own adaptation mechanisms (Hernández et al., 2014; Ostolaza, 2014); however, rhizospheric soil bacteria also influence plant growth, which is why they are called plant growth-promoting rhizobacteria or PGPR (Fonseca et al., 2016).
Drought and salinity are significant problems in agriculture, and both generate stress due to water deficit in plants, which affects plant growth and development (Forni et al., 2016); however, PGPR such as Actinobacteria with 1-aminocyclopropane-1-carboxylate enzyme activity (ACC deaminase) decrease drought stress (Abbasi et al., 2020), as well as salinity stress (Yoolong et al., 2019). It has been reported that the inoculation of Actinobacteria with ACC deaminase activity under salinity stress increased plant height and root length of Triticum durum L. “wheat” (Djebaili et al., 2021), increased root length, biomass weight and grain yield of Oryza sativa "rice" (Kapadia et al., 2022), enhanced the yield of Glycine max "soybean" (Gao et al., 2022), and increased the growth, yield and biochemical characteristics (Rangseekaew et al., 2021; Gong et al., 2020), as well as the antioxidant activity catalase, peroxidase, ascorbate peroxidase and the content of proline and soluble amino acids (Nozari et al., 2022) of tomato plants.
In addition to the activity of the ACC deaminase enzyme, Actinobacteria solubilize inorganic phosphates, produce indole acetic acid (Djebaili et al., 2021), fix nitrogen (Kaur & Manhas, 2022), produce siderophores (Rangseekaew et al., 2021) and present enzymatic activity related to the control of phytopathogens (Djebaili et al., 2020; Gong et al., 2020). Bacteria with ACC deaminase enzyme activity can also constitute biofertilizers for agricultural crops in soils impacted by salinity. In this context, the Actinobacteria from the prickly pear rhizosphere have the potential to reduce drought stress, a problem generated by salinity; however, prior to their use, they must be isolated and characterized with the perspective of their application in crops of economic importance such as tomato.
For all these reasons, this research aimed to determine the potential of the Actinobacteria isolated from the prickly pear rhizosphere as tomato growth promoting under salinity conditions.
2. Materials and methods
2.1. Sampling location
The soil of the rhizosphere was collected from prickly pear plants, in Lambayeque, Peru. In the coastal area, Lambayeque has a temperate climate with moisture deficiency in all seasons of the year, with a maximum temperature of 31 °C, a minimum of 21 °C, and annual rainfall between 0-5 mm (SENAMHI, 2023).
The rhizospheric soil samples were collected (0.2 m from the base of the stem of the plants) at a depth of 0.15 - 0.20 m to reach the roots, which were cut together with the adhering soil. Likewise, 2 kg of root soil was collected to determine the physical and chemical characteristics in the Laboratorio Agrícola de Análisis de Suelo y Agua, CYSAG. The analysis showed that the soil is sandy loam, with an acidic pH (pH 5.31), slightly saline (EC =5.51 dS/m), very low level of CaCO3 (1.5%), medium level of organic matter (2.99%) and high levels of phosphorus (55 mg/kg) and potassium (350 mg/ kg).
2.2. Phenotypic identification of the actinobacteria isolated from the prickly pear rhizosphere
Actinobacteria were isolated from rhizospheric soil in oatmeal agar (0.85 M NaCl ~ 5% NaCl) supplemented with 100 ug/mL of the antifungal Nystatin (Horstmann et al., 2020). After 7 days of incubation at 30°C, the typical colonies of Actinobacteria were selected and grouped considering the color of the aerial and substrate mycelia (Damodharan et al., 2018). For microscopic characterization (Olympus CX23 Microscope, Evident Corporation), the Actinobacteria were seeded on oatmeal agar and a cover slip was introduced at an angle of 45° with respect to the agar surface. After 5 days of incubation at 30 °C, the coverslips were placed on slides with two drops of crystal violet for microscopic observation of the vegetative and aerial mycelia and the reproductive structures (Ruiz, 2021).
2.3. Selection of Actinobacteria with ACC deaminase activity
The Actinobacteria were seeded in duplicate using Dworkin & Foster (DF) minimal medium with the nitrogen sources ACC (5 mM, SIGMA-Aldrich) and ammonium sulfate (2g/L) as positive control, both supplemented with 0.85 M NaCl. After 5 days of incubation at 30 °C, the absorbance of the cultured media was determined in a visible light spectrophotometer (Model Tenso Med NV-203) at 405 nm. Bacteria with ACC deaminase activity were those that developed using ACC as nitrogen, and the absorbance of the cultured medium was similar to or greater than the positive control (Rangseekaew et al., 2021).
2.4. Effect of Actinobacteria on the germination of tomato seeds under salinity conditions
The effect of Actinobacteria on seed tomato cultivar Río Grande germination under salinity stress was determined in Petri dishes conditioned with filter paper moistened with distilled water (control without inoculation EC= 0 dS/m) and a solution of 80 mM NaCl (EC= 6.94 dS/m). The inoculum was obtained with the DF minimal medium (0.85 M NaCl and 3 mM ACC), cultured with the bacteria for 6 days, centrifuged for 5 minutes, rinsed three times with a NaCl solution (0.85 M NaCl), resuspended in the same solution and with a concentration of 108 conidia/mL (Zhang et al., 2019).
The seeds were inoculated with the bacteria for 10 hours including distilled water and NaCl (both without inoculation) as controls. After 30 minutes of drying, the seeds were deposited in Petri dishes (40 seeds per dish). Seeds with the emerged radicle (2 mm) were considered germinated, and the percentage of germination was calculated every 3 days (Amna et al., 2019):
2.5. Comparative effect of three Actinobacteria on tomato growth, yield and chlorophyll content in non-saline and saline soils
In two trials, the effect of three Actinobacteria on the development of tomatoes in soils with and without salinity was determined, under a completely randomized design (CRD) with five treatments corresponding to control without inoculation (TI), a chemical control without Actinobacteria (T2), Actinobacteria 1 (T3), Actinobacteria 2 (T4) and Actinobacteria 3 (T5) in each test.
Tomato cultivation and inoculation of three Actinobacteria with ACC deaminase activity were carried out in the greenhouse of the Universidad Nacional Pedro Ruiz Gallo (UNPRG) in Lambayeque, from November 2019 to March 2020, with maximum temperatures of 28 - 30 °C, minimum 20 - 22 °C, and average 24 - 26 °C, according to the record of the UNPRG Meteorological Station in Lambayeque.
The inoculum was obtained with the DF minimal medium (0.85 M NaCl and 3 mM ACC), cultured with the bacteria, centrifuged, resuspended in a NaCl solution (0.85 M NaCl) and with a concentration of 108 conidia /mL (Zhang et al., 2019). The tomato seeds were placed in polyethylene bags (2.5 x 8.0 cm) where they were mixed with 0.5 mL of the bacterial inoculum for 9 hours, considering distilled water for the control without inoculation. After 30 minutes of drying, the seeds (five) were sown in cups with 425 g of a mixture of river sand and humus (1:1) previously sterilized in the autoclave at 121 °C and 1 atmosphere of pressure for 3 hours (Escobar et al., 2011).
After 40 days of sowing, three seedlings were eliminated and the two most vigorous remained, which were transplanted into clay pots with 3 kg of non-saline (EC = 1.40 dS/m and PSI = 0.68%) and saline (EC = 10.28 dS/m and PSI = 9.58%) soils according to the corresponding treatment. Before transplanting, the roots of the seedlings were immersed in 300 mL of the bacterial inoculum. Dechlorinated water was applied as the control without inoculation. On the other hand, chemical control was used to compare the effect of chemical fertilizers with biological fertilizers (Actinobacteria). This chemical control was composed of fertilizer with 46% nitrogen as urea, 46% phosphorus as diammonium phosphate, and 50% potassium as potassium sulfate (50% K).
For 60 days, after transplanting, and at 15-day intervals, the height and number of leaves of the plants were recorded. At 45 days, the relative chlorophyll content was determined using a chlorophyllometer (Minoltan Spad 502 plus) and the total content was calculated using the equation used by Jiang et al. (2017):
Total chlorophyll (mg/g) = [0.0306 (X) + 0.1443]
X is the value (SPAD) read from the chlorophyll meter. At 120 days after transplanting, the fruits were harvested in the ripening state to determine the number and weight of the fruits. Next, the length and weight of the root biomass and the weight of the aerial biomass were recorded, and the percentages of increase were calculated. Likewise, in the CYSAG laboratory, the ionic content was determined, for which potassium and sodium concentrations were quantified and the K+/Na+ rate was calculated in the leaves and roots of the plants with the highest yield in saline soil.
2.6. Statistical analysis
The values of the growth and yield parameters were analyzed to establish their normality and homoscedasticity (Shapiro - Wilk and Bartlett, p > 0.05), to determine the differences between treatments (Analysis of variance, p < 0.05) and significance between them (Tukey's multiple comparisons test, p < 0.05). SPSS statistics V22.0 (IBM) was used for all statistical analyses.
3. Results and discussion
3.1. Genera of Actinobacteria isolated from prickly pear rhizosphere soil
In 87.03% of the prickly pear rhizosphere soil samples, 150 pure cultures of Actinobacteria were obtained, (strains named by correlative Arabic numerals according to the order of isolation), grouped into 36 morphotypes, according to macroscopic and microscopic characteristics. Streptomyces (46.67%), Nocardia (34.0%), Micromonospora (6.00%), Pseudonocardia (4.67%), Nocardiopsis (3.33%), Nocardioides (3.33%) and Streptoverticillium (2.0%) were identified (Figure 1, and Figures S1, S2 and S3 of Supplementary Material). The phenotypic identification coincides with Ruiz (2021), Qi et al. (2019) and Fonseca et al. (2016). The rhizospheric microorganisms of cacti constitute a source of genes that provide them the characteristics that allow survival in environments with drought because of lack of rain or salinity (Bustillos et al., 2017).
3.2. Selected actinobacteria from the prickly pear rhizosphere with ACC deaminase activity
9.33% of prickly pear rhizospheric Actinobacteria presented ACC deaminase activity evidenced by development in minimal Dworkin & Foster media with ACC and ammonium sulfate as nitrogen sources with similar absorbance between them or a higher absorbance in the medium with ACC. The positive results of this study were higher than those obtained by Ruiz (2021) of Actinobacteria isolated from Echinopsis pachanoi "San Pedro" (7.69%) and lower than those obtained by Horstmann et al. (2020) in Fabaceae (45.4%) and Nozari et al. (2021) in wheat, melon and Brachiaria sp. (60%). ACC deaminase activity is a PGPR mechanism that favors plants, especially under unfavorable conditions (Abbasi et al., 2020; Jaemsaeng et al., 2018).
3.3. Effect of Actinobacteria on the germination of tomato seeds cv. Rio Grande under salinity stress
The germination percentage of tomato seeds under salt stress was from 5.83% (Streptoverticilium sp. strain 1) to 26.68% (Streptomyces sp. strain 21) at 6 days; 7.50% (Streptoverticillium sp. strain 1) to 28.33% (Streptomyces sp. strain 21) at 9 days; 5.00% (Streptomyces sp. strain 105) to 25.00% (Streptomyces sp. strain 10) at 12 days and 5.83% (Streptomyces sp. strain 49) to 24.18% (Streptomyces sp. strain 10) at 15 days. In the control without inoculation (distilled water), the germination percentages were 25.83% (6 days); 20.83% (9 days); 22.50% (12 days) and 19.18% (15 days); and in the NaCl control, the values were 4.18; 5.0; 2.50 and 4.18% respectively. This data coincides with the report by Amna et al. (2019), who reported that the inoculation of Bacillus spp. with ACC deaminase activity increased wheat seed germination up to 69.32% under EC= 16 dS/m. The promotion of seed germination under salinity by bacteria is associated with an increase in the efficiency of water use (Li et al., 2021) and the activity of the enzyme α amylase (Amna et al., 2019; Ameur & Ghoul, 2014), as well as the bacterial metabolites auxins and gibberellins (Aly et al., 2012) and volatile organic compounds (Dias et al., 2017).
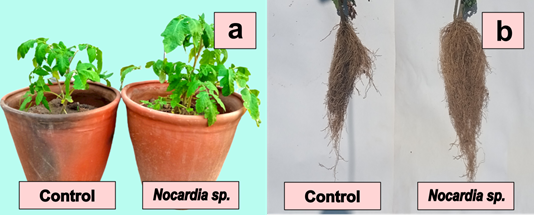
Figure 2 Solanum lycopersicum L. inoculated with Nocardia sp. strain 13 in a non-saline soil, plants after 70 days (a) and roots after 120 days (b) of sowing.
3.4. Comparative effect of three Actinobacteria on growth, yield and chlorophyll content in tomato
Actinobacteria increased growth parameters (height, number of leaves, weight of aerial biomass, length and weight of roots) and yield (number and weight of fruits) of tomato plants in non-saline (Figure 2) and saline soils (Figure 3) significantly. The high concentration of salts (EC= 10.28 dS/m) in the soil impacted the development of the plants negatively; however, the inoculation of the Actinobacteria improved the investigated characteristics, reaching the highest percentages of increase in the saline soil.
In the non-saline soil, an increase rate of 15.83% (15 days) was reached; 55.12% (30 days); 62.63% (45 days) and 52.72% (60 days) at altitude; 69.05% (number of sheets); 144.0% (aerial biomass); 91.43% (root length); 109.06% (root weight); 61.11% (number of fruits) and 76.95% (weight of fruits). In the saline soil, an increase rate of 70.45% (15 days) was reached; 80.0% (30 days); 88.98% (45 days) and 84.56% (60 days) at height; 96.30% (number of sheets); 201.35% (aerial biomass); 173.77% (root length); 100.0% (root weight); 150.0% (number of fruits) and 173.14% (weight of fruits) (Figure 4and5). This agrees with the results of Gong et al. (2020), who reported increases of 21.7% (root length); 42.4% (height) and 67.4% (weight of aerial biomass) in the plants inoculated with Streptomyces sp. in the saline soil, compared to 16.2; 15.8 and 52.8%, respectively, in the non-saline soil.
The increase of the values in the development parameters of the tomato plants by the Actinobacteria with ACC deaminase activity in the saline soil coincides with the reports of Rangseekaew et al. (2021), Gong et al. (2020) and Damodharan et al. (2018). These investigators determined that inoculation of Streptomyces spp. favored the growth of tomato plants developed with 150, 180 and 200 mM NaCl, respectively. An increase in height, weight of aerial biomass, root length, chlorophyll content, potassium and decrease in accumulated sodium and hydrogen peroxide content were reported.
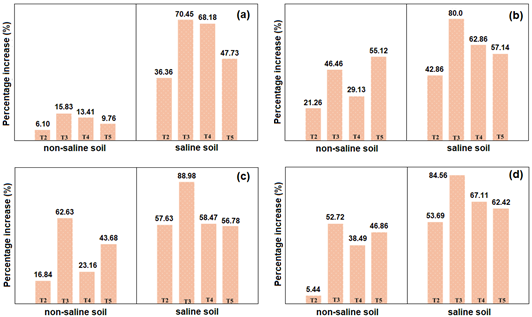
Figure 4 Percentage increase in the height of Solanum lycopersicum L. in non-saline and saline soils at 15 days (a), 30 days (b), 45 days (c) and 60 days (T2=chemical, T3= Streptomyces sp. strain 21, T4=Streptomyces sp. strain 10, T5=Nocardia sp. strain 13).
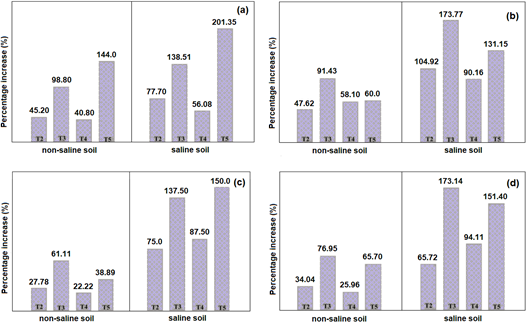
Figure 5 Percentage increase in aerial biomass (a), root length (b), number (c) and fruit weight (d) of Solanum lycopersicum L. in non-saline and saline soils (T2=chemical, T3 =Streptomyces sp. strain 21, T4=Streptomyces sp. strain 10, T5=Nocardia sp. strain 13).
The rhizospheric Actinobacteria of prickly pear increased the length of the roots up to 173.77% and a value greater than 88.0% of the increase in the height of the tomato plants at 45 days. This agrees with the results of Puppala et al. (2019) who reported average increases of 50% and 5% in root length and plant height, respectively, and attributed the superiority of the values in the roots to indole acetic acid produced by Streptomyces sp. strain NCIN inoculated into tomato seeds.
The Actinobacteria increased up to 144% of the aerial biomass and 109% of the root biomass, a difference that can be explained by Miceli et al. (2021), who concluded that the effect of PGPR such as Actinobacteria with ACC deaminase activity becomes evident when conditions are unfavorable; in this study, aerial biomass received more benefit from bacterial inoculation.
Tukey's multiple comparison test showed that in the non-saline soil, the highest value in height corresponded to Streptomyces sp. strain 21 (45 and 60 days) and Nocardia sp. strain 13 (30 days), in aerial biomass to Nocardia sp. strain 13, in root length and weight to Streptomyces sp. strain 21, in the number of fruits to Actinobacteria and the chemical control and in the weight of fruits to Streptomyces sp. strain 21 (Table 1). In the saline soil, the highest height values were reached with Streptomyces sp. strain 21 (45 and 60 days), in the number of leaves and fruits with the Actinobacteria and the chemical control, in the aerial biomass and weight of roots with Nocardia sp. strain 13, and root length and fruit weight with Streptomyces sp. strain 21 (Table 2).
Regarding the content of foliar chlorophyll in the non-saline soil, 1.37-1.52 mg/g were reached with the Actinobacteria, compared to 1.38 (chemical control) and 1.24 mg/g (control without inoculation). The highest values were reached with Nocardia sp. strain 13 and Streptomyces sp. strain 21, with significant differences compared to the other treatments. In the plants developed in the saline soil, the chlorophyll content was 1.20 - 1.37 mg/g with the Actinobacteria compared to 1.31 mg/g (chemical control) and 1.03 mg/g (control without inoculation). The highest values corresponded to plants with Streptomyces sp. strain 21, Nocardia sp. strain 13 and the chemical control, with significant differences compared to the control without inoculation and Streptomyces sp. strain 10 (Figure 6). The increase in chlorophyll content in tomato plants inoculated with Actinobacteria was previously reported by Djebaili et al. (2020) in non-saline soil and Rangseekaew et al. (2021) and (2022) in saline soil. In this regard, Aini et al. (2021) demonstrated an 8-13% increase in the chlorophyll content of tomato plants with one to four applications of a bacterial consortium that included Streptomyces sp. Likewise, Gong et al. (2020) reported that Streptomyces sp. increased total chlorophyll in tomato plants, but without significant differences with the control without inoculation.
Table 1 Tukey's multiple comparison test of growth and yield parameters of Solanum lycopersicum plants inoculated with Actinobacteria in a non-saline soil
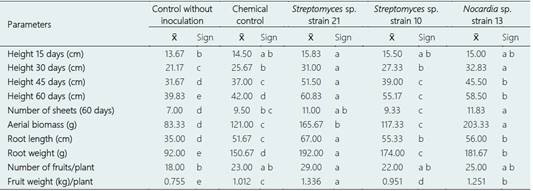
Table 2 Tukey's multiple comparison test of growth and yield parameters of Solanum lycopersicum plants inoculated with Actinobacteria in a saline soil
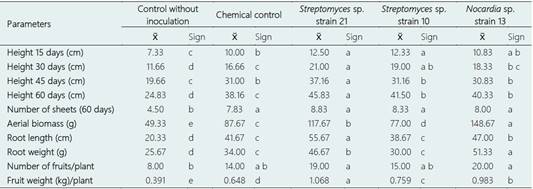
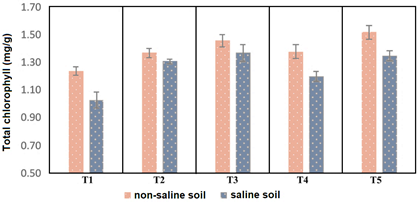
Figure 6 Mean leaf chlorophyll content in Solanum lycopersicum plants inoculated with Actinobacteria in non-saline and saline soil.
Table 3 Potassium, sodium and K+/Na+ rate in leaves and roots of Solanum lycopersicum L. plants inoculated with Streptomyces sp. strain 21 in a saline soil

Salinity decreases chlorophyll content and therefore impacts plant photosynthesis. Rangseekaew et al. (2021) showed that Dermacoccus spp. increased photosynthetic pigment, which favored fresh and dry aerial biomass and root length. On the other hand, Rangseekaew et al. (2022) determined a 64% reduction in the total foliar chlorophyll content in tomato plants grown with 150 mM NaCl; however, the inoculation of D. abyssi increased the photosynthetic pigment. These researchers concluded that photosynthesis was enhanced by Actinobacteria, which reduced salinity-induced hydrogen peroxide.
The biochemical analysis of the leaves from the plants grown under salinity was used to explain the beneficial effect of the Actinobacteria. The ionic content of the tomato plants was affected by the inoculation of Streptomyces sp. strain 21 in saline soil. Sodium content decreased, but potassium content and the K+/Na+ rate increased in the leaves and roots of plants inoculated with Streptomyces sp. strains 21 compared to the control without inoculation (Table 3). The decrease in sodium content and the increase in potassium content and K+/Na+ rate in the leaves and roots of plants inoculated with Streptomyces sp. strain 21 under salinity showed that Actinobacteria help in the maintenance of ionic homeostasis. Similarly, Gong et al. (2020) showed that sodium increased, and potassium decreased in tomato leaves under conditions of 200 mM NaCl; however, the inoculation of Streptomyces sp. KLBMP508 favored sodium exclusion and increased potassium content and K+/Na+ ratio. In this context, the beneficial effect of Streptomyces spp. strains 10 and 21 and Nocardia sp. strain 13 in the growth and yield of tomato showed that these bacteria can be used in the formulation of biofertilizers for the management of plants under salinity.
Additionally, when plant growth-promoting Actinobacteria are inoculated to agricultural fields with salinity, they establish beneficial interactions with the resident microbial community, as demonstrated by Hu et al. (2020), who found a positive correlation between Streptomyces sp. TOR3209 and the beneficial rhizobacteria Enterobacter sp., Arthobacter sp., B. subtilis, and B. velezensis. Likewise, Actinobacteria that colonize the root system improve the nodulation of plants (Said, 2022) and reduce the probability of infection by phytopathogens (Gao et al., 2022). Although the effect of the use of these actinobacteria on the resident bacterial community of tomatoes was not evaluated, it can be assumed that most of these effects are positive based on all this previous evidence.
4. Conclusions
The rhizospheric Actinobacteria of Opuntia sp. with ACC deaminase activity increased the growth and yield of seedlings with increases of 88.98% (height); 96.30% (number of sheets); 201.35% (aerial biomass); 173.77% (root length); 100.0% (root weight); 150% (number of fruits) and 173.14% (weight of fruits), as well as 1.37 mg/g in the chlorophyll content of tomato plants in the saline soils. Likewise, Streptomyces sp. strain 21 decreased sodium content and increased potassium content and K+/Na+ rate in the leaves and roots of tomato plants with the highest yield in saline soil (1.068 kg/plant), evidencing the potential of this Actinobacteria to reduce salinity stress and constitute a fertilizer for tomato cultivation. It is recommended to characterize the properties of plant growth-promoting Actinobacteria using biochemical methods, the impact of its use on the resident bacterial community, the identification of species using molecular techniques, and to continue the search for new candidate strains that favor tomato growth under saline stress conditions.