1. Introduction
Worldwide, there is a great interest in using plant extracts enriched in bioactive compounds for the synthesis of nanoparticles (NP), as a climate intelligent practical alternative for current protocols based on toxic chemicals (Huq et al., 2022; Akter & Huq, 2020; Jamkhande et al., 2019; Jain & Mehata, 2017; Gómez et al., 2016). Specifically, silver nanoparticles (AgNPs) are of great interest thanks to their antioxidant (Huq & Akter, 2021; Huq, 2020; El-Naggar et al., 2017; Aboul-Enein et al., 2016; Kokila et al., 2015) and antibacterial activities (Huq & Akter, 2021; Huq, 2020; Pawliszak et al., 2019; Jemilugba et al., 2019). On the other hand, cellulose microfibers (CMF) are also important raw material for the production of pharmaceutical drugs, textiles and food, among others (Kassab et al., 2020a; Kumar et al., 2020; Lessa et al., 2020; Kassab et al., 2019; Trache, 2018; Rajinipriya et al., 2018; Grishkewich et al., 2017), since their morphology, chemical structure and appearance can be modified depending on the extracting procedure (Risite et al., 2022; Ait Benhamou et al., 2021; Kassab et al., 2020b; Kumar et al., 2020; Arumai Selvan et al., 2018; Thomas et al., 2018; Rajinipriya et al., 2018; Trache et al., 2017; Deepa et al., 2015).
In banana-producing countries, a large amount of crop waste can be used to produce silver nanoparticles and cellulose microfibers due to their high content of bioactive compounds and fiber content (Tibolla et al., 2018; Harini et al., 2018). The peel of bananas could be a suitable candidate for the synthesis of AgNPs based on its high content of bioactive compounds such as tannins flavonoids and saponins (Vu et al., 2018; Prakash et al., 2017; Aboul-Enein et al., 2016; Padilla-Camberos et al., 2016; Amutha & Selvaku-mari, 2016). On the other hand, the rachis of bananas is known to be rich in cellulose fibers, converting this plant organ into an excellent provider of CMF for the industry (Lessa et al., 2020; Jirón et al., 2020; Cecci et al., 2020; Rajinipriya et al., 2018). Therefore, the aim of this research was to design a protocol for the synthesis of AgNPs and CMF to be combined into a micro-composite using banana waste as the prime material. The designing of a suitable protocol for the synthesis of banana peel based AgNPs included the determination of the pH and temperature ranges of the reaction media for optimal yield (Sengupta & Sarkar, 2022; Kumar et al., 2017; Kokila et al., 2015; Ibrahim, 2015).
The effectiveness of the AgNPs and micro-composite was analyzed in terms of their antimicrobial and antioxidant properties.
2. Materials and methods
2.1 Plant material
Bunches of organically grown Musa acuminata var. “Williams” bananas at the maturity stage degree 4 (Madan et al., 2014) were purchased at local markets in the Province of Manabí, Ecuador. At the laboratory, rachis and peels were removed and cut into small pieces to be thoroughly washed with tap water to remove the latex and rinsed with distilled water for 5 minutes. Thereafter, the excess water was drained and the pieces were soaked in 2% citric acid for 15 minutes, next, pieces were rinsed 6 times with distilled water, then, drained and dried for 3 hours at room temperature for later use (Figure 1).
2.2 Preparation of extracts from banana peels and synthesis of silver nanoparticles
The banana peels were dried in the oven (Memmert SLM 500) at 40 ºC for 7 days until constant weight, ground in a mortar and sifted through a 28-mesh (Cole Parmer YV-59980-08) sieve a fine powder. Ten grams of the fine powder were extracted and mixed with 100 ml of (a) distilled water, (b) ethanol, (c) methanol (d) water-ethanol (1:1) and (e) water-methanol (1:1) in 250 ml Erlenmeyer flasks with constant agitation in an Orbital Shaker (VWR Standard 3500) for 10 min. Extracts were kept for 24 h at room temperature, followed by filtration through a paper Whatman # 1 and refrigerated at 4 °C for later use. Peel extracts were qualitatively analyzed for compounds tannins, saponins and flavonoids content as described by Padilla-Camberos et al., (2016). The water-ethanol extract with the greatest content of bio-compounds was selected for further synthesis of the AgNPs. The content of biocompounds was determined following the proto cols for tannins (AOAC.952.03; AOAC, 2000), saponins (Ridout et al., 1991) and flavonoids (Mabry et al., 1970) (Figure 1).
For the synthesis of AgNPs, 5 ml of 1 mM silver nitrate AgNO3 (Sigma-Aldrich) were mixed with 52, 156, 260 or 520 µl aliquots of the water-ethanol peel extract following the protocol described in Kokila et al. (2015). To assess the effects of pH and temperature, the pH of the AgNO3·solution was adjusted to 6, 8, or 10 with 1% NaOH, and reactions were carried out at 27, 40, 50, or 60 °C in a temperature-controlled water bath (TECNAL TE-0541/1) for 30 minutes in darkness. The synthesized AgNPs were stored in amber jars at 4 °C. The visible/UV absorbance of the AgNPs was determined in a UV-visible spectrophotometer (Thermo Scientific Genesys 10s series), while, the physical characteristics of the AgNPs were determined in a scanning electron microscope (TESCAN Mira3 XMU, USA Inc.) (Figure 1).
2.3 Preparation of banana rachis for the synthesis of cellulose micro-fibers (CMF)
Pieces of the rachis were dried in the oven at 100 °C for 3 days, until constant weight, ground in a mortar, and sifted through a 28-mesh (Cole Parmer YV-59980-08) sieve to obtain a fine powder. CMF were isolated from the rachis powder following the protocol described by Deepa et al. (2015). Briefly, 7 g of rachis powder with 70 ml of 2% NaOH was autoclaved (All American 75X-120V) for 1 h at 20 lbs of pressure. The mixture was allowed to reach room temperature, then the pellet was filtered and washed 3 times with distilled water to neutralize the pH. The cellulose fibers (CF) were bleached in 15 ml of 1% NaClO2 pH 2 for every gram of fiber, in water bath (TECNAL TE-0541/1) at 70 °C, next the CF was filtered and repeated several times until the fibers were totally white. Then, the bleached cellulose fibers were filtered, later, washed 3 times with distilled water, and oven (Memmert SLM 500) dried for 2 h at 60 °C (Figure 1).
One gram of CF was subjected to acid hydrolysis with 15 ml 5%, 7% or 9% oxalic acid (C2H2O4) in an autoclave (All American 75X-120V) at 20 lbs, for 3 h. Then, the pellet was filtered and rinsed 3 times with distilled water to neutralize the pH. Finally, the cellulose micro-fibers (CMF) were suspended in water and then subjected to stirring for 6 h in an orbital shaker (VWR Standard 3500) and saved for later use. The physical characteristics of CMF were determined in a scanning electron microscope (SEM) (TESCAN Mira3 XMU, USA Inc.) (Figure 1).
2.4 Micro-composite (AgNPs and CMF)
The micro-composite was obtained by combining 5 g of dried CMF and 95 ml of AgNPs under constant agitation for 6h according to the protocol described in Dong et al. (2013) (Figure 1).
2.5 Antioxidant capacity of AgNPs, CMF and micro-composite
Antioxidant properties of the AgNPs and micro-composite were determined using the 2,2-Diphenyl-1-picrylhydrazyl (DPPH) test (Aboul-Enein et al., 2016; Padilla-Camberos et al., 2016). For this, aliquots of 50 µl of AgNPs or micro-composite were mixed with 2 ml of 0.1 mM DPPH, then, incubate in darkness for 30 min to ensure complete neutralization of the radical. The absorbance of the reaction was measured at 520 nm in a spectrophotometer (Thermo Scientific Genesys 10s series). The percentage of inhibition was determined by the formula (1) (Aboul-Enein et al., 2016; Padilla-Camberos et al., 2016):
2.6 Antibacterial capacity of AgNPs, CMF and micro-composite
The antibacterial activity of AgNPs and micro-composite was determined using the agar-plate disk diffusion method (Coyle et al., 2016). With the Gram-negative (Escherichia coli “ATCC: 25922”) and Gram-positive (Staphylococcus aureus “ATCC: 25923”) as reference bacterial species. Aliquots of 100 µL of the inoculum containing 105 - 106 CFU/mL of each bacterial strain were spread uniformly on the agar surface with the help of a glass L-rod. The petri-dish divided into four parts previously inoculated with E. coli and S. aureus, were added sterile filter paper discs 5 mm with 10 µl of the extract, AgNPs, micro-composite and antibiotic Ciprofloxacin. The results were obtained by incubating the sample for 24 hours at 37 °C.
2.7 Statistical analysis
Statistical analysis was performed using Statgraphics Centurion XVI software. All experimental data were expressed as the statistical mean ± standard deviation and derived from triplicate assays. The Tukey HSD test sample was applied to assess the statistical significance of the data. A p-value less than 0.05 was considered significant.
3. Results and discussion
3.1 Selection of the plant extract
Present results confirmed the water-ethanol extract of banana peels as the most favorable for the synthesis of AgNPs due to its greatest content of bio-compounds, when compared to the rest of the extracts (Table 1). Analyses revealed 1.6 g kg-1 tannins, 452 mg Kg-1 flavonoids and 16.6 g kg-1 saponins in the water-ethanol extract in accordance, with previous publications by (Prakash et al., 2017; Aboul-Enein et al., 2016; Amutha & Selvakumari, 2016; Padilla-Camberos et al., 2016).
Table 1 Qualitative analysis of banana peel extracts (AAA)
SAPONINS | TANNINS | FLAVONOIDS | |
---|---|---|---|
Water | - | - | - |
Ethanol | - | ++ | - |
Methanol | - | ++ | - |
Water-ethanol | ++ | ++ | ++ |
Water-methanol | ++ | + | ++ |
(++) abundant, (+) medium, (-) absence.
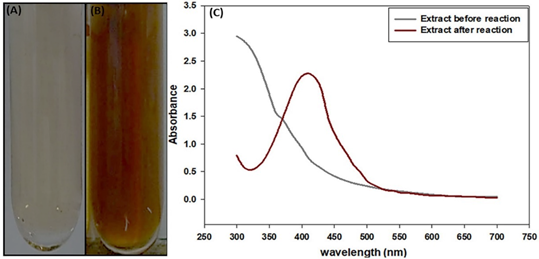
Figure 2 Reaction of synthesis of nanoparticles: (A) extract before reaction, (B) extract after reaction and (C) Graph of UV-Vis spectrophotometry of extract before reaction and after reaction.
Table 2 Micro-cellulose fibers yield of the rachis
TREATMENT OXALIC ACID | INITIAL CELLULOSE (g) | MICRO-CELLULOSE (g) | YIELD (%) | AVERAGE OF YIELD |
5 | 2.87 ± 0.01 (a) | 1.04 ± 0.13 (a) | 36.41 ± 4.68 (a) | 36.12 ± 2.82 |
7 | 2.88 ± 0.01 (a) | 1.01 ± 0.11 (a) | 35.01 ± 3.70 (a) | |
9 | 2.87 ± 0.01 (a) | 1.06 ± 0.01 (a) | 36.93 ± 0.49 (a) |
3.2 AgNPs synthesis and properties
The reduction of silver ions into AgNPs was evidenced by the color change from yellow to dark brown in the reaction mixture (Figure 2A,B) (Sengupta & Sarkar, 2022; Moshahary & Mishra, 2021; Varadavenkatesan et al., 2019; Ahmad et al., 2003), displaying a peak of absorbance at 410 nm (Figure 2C) (Moshahary & Mishra, 2021; Varadavenkatesan et al., 2019; Ahmad et al., 2003), that coincide with the plasmon surface resonance excitation of the AgNPs (Sengupta & Sarkar, 2022; Moshahary & Mishra, 2021; Varadavenkatesan et al., 2019; Ahmad et al., 2003).
Moreover, maximum absorption of the AgNPs at 410 nm (Table 3) was detected in the reaction mixture containing an aliquot of 156 µl of the banana peel water-ethanol extract (Figure 3A), These results point toward the importance of the calibration of the protocol for optimal yield of AgNPs.
On the other hand, the basic pH 10 of the AgNO3 solution promoted the synthesis of the AgNPs above values at pH 8 (Figure 3B), in agreement with previous findings (Ibrahim, 2015; Kokila et al., 2015). Interesting to note the hindering effects of pH 6 on the synthesis of AgNPs. It is also known, the interaction between the pH and the shape and size of the nanoparticles (Huq et al., 2022; Kumar et al., 2017). Furthermore, incubation of the reaction mixture at 60 °C proved to be the optimum for the synthesis of the AgNPs (Figure 3C), similarly at the results by Moshahary & Mishra (2021) (Table 3).
3.3 Isolation of Micro-cellulosic fibers (CMF)
Designed protocols had a yield of 36.12 ± 2.82% of CMF, regardless of the percentage of oxalic acid used for the acid hydrolysis (Table 2). Therefore, the lowest acid concentration was selected as the less environmentally contaminant. It is important to note that the obtained yield of CMF was higher than that reported by Deepa et al. (2015) in banana rachis (Table 3).
3.4 SEM analysis
The SEM image of AgNP showed uniform 24 nm diameter spherical nanoparticles (Figure 4A), in agreement with previous findings (Ibrahim, 2015) (Table 3). On the other hand, the size of isolated CMF oscillated between 350 - 50 µm with a diameter between 10 - 5 µm (Figure 4B). Previous studies it has been determined the morphology and dimension of the AgNPs and CMF varies among different sources and process to obtained (Table 3). (Huq et al., 2022; Sengupta & Sarkar, 2022; Risite et al., 2022; Moshahary & Mishra, 2021; Sankhla et al., 2021; Bahloul et al., 2021; Jirón et al., 2020; Cecci et al., 2020; Varadavenkatesan et al., 2019; Rajinipriya et al., 2018; Ibrahim, 2015; Tibolla et al., 2018; Harini et al., 2018).
3.5 Antioxidant properties of AgNO3 and the micro-composite
The AgNPs displayed 71.1 ± 0.45 antioxidant activity, 1.8-fold greater than the pure water-ethanol extract in the DPPH scavenging activity, the AgNPs was transferred to micro-composite (AgNPs + CMF) with 72.2 ± 0.38% of the activity. On the other hand, the scavenging activity of the extract water-ethanol was ascribed to the bioactive compounds present in the extract (Figure 5A) and in agreement to values recorded ethanol extracts peels of Musa ParadiseacaAboul-Enein et al. (2016).
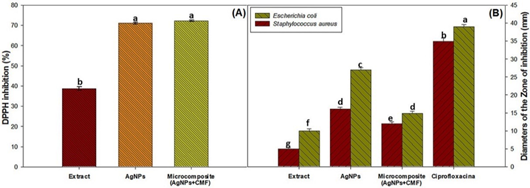
Figure 5 Biological characterization of the Extract, AgNPs, Micro-composite. A) DPPH inhibition (%), B) Bacterial inhibition.
Table 3 Morphology of AgNPs and CMF
AgNPs | |||||
---|---|---|---|---|---|
Plant | Part of Plant | Absorbance | diameter | shape | Reference |
Banana (Musa acuminata Williams) | Peel | 410 nm | 24 nm | Spherical | This study |
Banana | Peel | 423 nm | - | Spherical | (Sengupta & Sarkar, 2022) |
Banana Var. Kachkol | Peel | 413 nm | - | Spherical | (Moshahary & Mishra, 2021) |
Banana (Musa paradisiaca) | Peel | 433 nm | 23.7 nm | Spherical | (Ibrahim, 2015) |
Banana (Musa acuminata Cavendish) | Peel | 430 nm | 23-30 nm | Spherical | (Kokila et al., 2015) |
Ipomoea digitata | Fower | 412 nm | sub-100 nm | Spherical | (Varadavenkatesan et al., 2019) |
Fusarium oxysporum | Colony | 413 nm | 5-50 nm | - | (Ahmad et al., 2003) |
Prunus persica | Leaves | 440 nm | 40-98 nm | Spherical | (Kumar et al., 2017) |
CMF | |||||
Plant | Part of Plant | Yield (%) | Size | Diameter | Reference |
Banana (Musa acuminata Williams) | Rachis | 36.12 ± 2.82 | 350 - 50 µm | 10 - 5 µm | This study |
Banana | Rachis | 28.6 | - | 10 - 60 nm | (Deepa et al., 2015) |
Banana (Musa acuminata Cavendish) | Rachis | - | 20-50 µm | - | (Jirón et al., 2020) |
Banana (Musa x paradisiaca) | Pseudostems | - | - | 110 µm | (Cecci et al., 2020) |
Banana (Musa paradisiaca Terra) | Peel | 71.51* | 619.57 nm* | 4.65 nm* | (Tibolla et al., 2018) |
27.07* | 310.77 nm* | 2.89 nm* | |||
Banana | Peel | 55.48 ± 1.57 | 351 µm | 30 -19.2 µm | (Harini et al., 2018) |
Bract | 64.67 ± 2.36 | 431 µm | 45 - 15 µm | ||
Artemisia annua | Stems | 53 | - | 5 - 30 µm | (Risite et al., 2022) |
Sugarcane | Bagasse | 78.71 ± 7.87 | - | 4 - 35 nm | (Sankhla et al., 2021) |
Solanum melongena L | Stems | 54 | - | 13.6 µm | (Bahloul et al., 2021) |
*Range in dependent of the acid concentration used during hydrolysis.
3.6 Determination of antimicrobial capacity
This well-known antibacterial activity of the AgNPs (Huq et al., 2022) was confirmed in the present work. As shown in Figure 5B., AgNPs caused inhibition of E. Coli and S. aureus colony growth, brought about by the nanoparticle disruption of the bacteria outer membrane (Sengupta & Sarkar, 2022; Huq et al., 2022; Sharma et al., 2020). On the other hand, the micro-composite showed similarly activity of the AgNPs, that suggest us the AgNPs transferred the ability to the micro-composite, similarly at the results by Phan et al. (2019) and Salama (2017). Nevertheless, the observed inhibition of colony growth by AgNPs was lower than that elicited by the Ciprofloxacin.
4. Conclusions
Present results confirm the benefits of using water-ethanol extracts of banana peels and rachis for the synthesis of AgNPs and CMF to conform a micro-composite. The high yields of AgNPs and CMF as well as the effectiveness of the AgNPs and micro-composite as antioxidant and antibacterial support the use of these banana part as prime material for the industrial production. The micro-composite could be an environmentally friendly replacement in the packaging of the food for transport and storage, allowing to reduce the accumulation of petrochemical microplastics in nature. However, it is necessary to continue with the studies due to the possible effects of migration of AgNPs and CMF to food, as well as their impact on human health. Nanoparticles could be used as an additive to biopesticides in pathogen control in crops.