1. Introduction
Mining is one of the most destructive anthropogenic practices that occur in soil (Ezenne et al., 2019), producing alterations in soil’s physical, chemical, and biological properties (Kaya et al., 2015). These alterations are represented by an abiotic stress gradient (Rosli et al., 2018).
Abiotic stress gradient is understood as the factors that exert fundamental restrictions on the distribution, form, and function of sessile soil organisms (Puglielli et al., 2023). It also consists of all those foreign elements of the soil system or the existence of an unusual level that generates a harmful effect on soil organisms and their consumers (Egea et al., 2022).
Abiotic stress gradients are determined by analysis with physicochemical parameters (Kaya et al., 2015). The main drawback of this method is that it provides ephemeral data (Campos-Herrera et al., 2016) “at a given point and time” (Ezenne et al., 2019) and is expensive (Ouyang et al., 2021). However, in recent years, bioindicators (Murphy et al., 2023) that provide data by season (Šalamún et al., 2018) have been used since organisms have different life cycles (Darré et al., 2019), address the issues with using ephemeral data (Bongers & Esquivel, 2015).
Organisms such as protozoa, algae and fungi present some bioindicator characteristics (Fournier et al., 2022); however, they have some disadvantages such as being at low levels of the food chain, which is why they do not integrate the chemical, physical and biological properties of food resources (Gautam et al., 2022); these organisms also have short life cycles, which is why their populations are less stable and are subject to temporary fluctuations due to ephemeral releases of nutrients (Kooch & Ghaderi, 2023).
Solving these drawbacks are edaphic nematodes, which present suitable characteristics compared to other organisms (Gu et al., 2018) since nematodes are found in all types of soil, as long as there is moisture (Calvão et al., 2019), are abundant (Bal et al., 2017) and easy to identify (Aujoulat et al., 2019), and represent up to 5 trophic groups (Campos-Herrera et al., 2016).
Although there are studies of nematodes in agricultural soils that indicate soil quality, it is necessary to further study the bioindicator capacity of nematodes in other types of soil (Kabir et al., 2018) to provide an adequate determination of soil quality. Therefore, this research aims to evaluate the bioindicator behavior of edaphic nematodes in soils influenced by mining.
2. Materials and methods
2.1 Study area
The study area was located on the periphery (500 to 1500 m) of the artisanal mining community of Ollachea, located in the Minapampa area of the mining company Kuri Kullu SA, in the Puno region, Carabaya Province and Ollachea district (Figure 1), at 13°47′41″S, 70°28′17″ W and at an altitude of 3041 masl. This area has a temperate and warm climate with an average annual temperature ranging between 6 and 18 °C, with a total average annual rainfall between 790 and 1000 mm. The soils have a texture ranging from moderately coarse to fine with a pH that is slightly acidic with calcareous lithologi cal material present in shallow and steep soils.
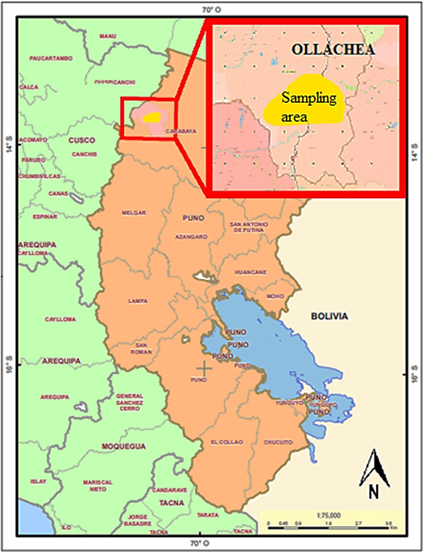
Figure 1 Location of the sampling area on the periphery of the mining community of Ollachea, Carabaya, Puno (Authors developed map in QGIS 3.24).
This study area has gold mineralization, which occurs free, disseminated and in micro veins with sulfide dissemination, the extraction activity that the area presents produces different events of remobilization of gold and sulfides as a result of metamorphism and tectonism, which enrich the areas. with contaminants that have detection limits in ppm and some in ppb (Mciver et al., 2022).
This study area was chosen based on the following criteria: presence of several types of edaphic ecosystems around the mining community and current land use suitable for agricultural and livestock activities that have a high influence of mining residues.
2.2 Sampling points and temporality
Four types of soil classified according to their use capacity were sampled: grassland (13° 47' 19" S, 70° 28' 00" W), clean crop - corn crop (13° 48' 39" S, 70° 27′ 07″ W), permanent crop - fig crop (13° 48′ 15″ S, 70° 28′ 30″ W) and forestry - eucalyptus (13° 47′ 01″ S, 70° 28′ 49″ W); in 100 m2 for each type of soil, between 500 and 1500 m away from the mine. The sampling was carried out in 1 rainy season and 1 dry season of 2021 to ensure a good representativeness of sampled nematodes (Achicanoy et al., 2012).
2.3 Sample collection
In the pasture and maize crop soils, zigzag sampling was used (Sechi et al., 2018); in the fig and eucalyptus crop soils, star-shaped sampling was used (Kaya et al., 2015). The extraction of the samples was performed with a cannula auger with a 4 cm diameter at a depth of 20 cm (Piedra, 2015). The sampling consisted of 20 subsamples and 3 repetitions for each type of soil (Coyne et al., 2007); the samples were placed and labeled in black polyethylene bags.
2.4 Measurement of edaphic, agrochemical, and heavy metal parameters
For each of the sampling points, 7 edaphic parameters were analyzed: cation exchange capacity (saturation method with ammonium acetate), calcium carbonate (NOM-021-SEMARNAT-2000 Item 7.1.1 method AS-07), conductivity (ISO 11265: 1994 Cor1: 1996), soil moisture (gravimetric method), organic matter (NOM-021-SEMARNAT-2000 Item 7.1.1 method AS-07), pH (EPA SW 846 method 9045 D, revision 4), and texture (sand, silt, and clay) (Robinson's pipette method); 9 nutritional parameters were analyzed (EPA method 200.7 Rev. 4.4 EMMC version/1994): boron (ppm), phosphorus (ppm), manganese (ppm), potassium (ppm), zinc (ppm), sulfur (meq/ 100 g), Al+ 3 + H+ (meq/100 g), calcium (meq/100 g), and magnesium (meq/ 100 g); 26 heavy metals were analyzed (EPA method 3050B/method 200.7 Rev. 4.4 EMMC version/1994): antimony (mg/kg), arsenic (mg/kg), barium (mg/kg), beryllium (mg/kg), bismuth (mg/kg), cadmium (mg/kg), cerium (mg/kg), cobalt (mg/kg), chromium (mg/kg), cesium (mg/kg), copper (mg/kg), tin (mg/kg), strontium (mg/kg), iron (mg/kg), lithium (mg/kg), mercury (mg/kg), molybdenum (mg/kg), nickel (mg/kg), silver (mg/kg), lead (mg/kg), selenium (mg/kg), silicon (mg/kg), thallium (mg/kg), titanium (mg/kg), uranium (mg/kg), and vanadium (mg/kg); and finally, 2 microbiological parameters were analyzed: microbial biomass carbon (Anderson & Domsch, 1978; Beck et al., 1995; Höper, 2006) and edaphic respiration (alkali trap).
2.5 Determination of edaphic nematodes
To obtain representativeness of active nematodes, the modified Whitehead tray method was used, and to obtain representativeness of inactive forms and slow nematodes, the modified centrifugation-flotation method was used (Hernández-Ochandia et al., 2016). With an Olympus stereoscope of 0.8 to 4.5 X magnification, nematodes were identified at the taxonomic level of genus. The identification of the specimens was performed based on taxonomic keys (Guerrero, 2017).
Determination of the abiotic stress gradient
The abiotic stress gradient was determined through a modification of the methodology proposed by Touron-Poncet et al. (2014), in which all the physicochemical variables (edaphic parameters, nutritional parameters, agrochemicals, and heavy metals) measured at all sampling points were standardized so that the data were comparable, and then, a principal component analysis (PCA) was performed. Exclusion of uncorrelated variables was determined by the variance inflation factor (VIF ≤ 10). The first axis was selected as the abiotic stress gradient, with this axis being the component that explained the highest percentage of variance of the PCA.
2.6 Determination and analysis of the relationship between nematodes and the abiotic stress gradient
The values of the abiotic stress gradient parameters were standardized between 0 and 1. Next, canonical correlation analysis (CCA) was performed to determine the correlation of nematodes and the abiotic stress gradient.
2.7 Data processing
All statistical analyses (PCA, CCA, and significance verification analysis) were performed using the statistical program RStudio (version 4.0.2), an active version of the R community, which is an open source data analysis software that is currently one of the most efficient and innovative programs being used for scientific studies (R Development Core Team, 2016).
3. Results and discussion
3.1 Determination of the abiotic stress gradient
For the rainy season, the 44 variables obtained a VIF ≥ 10; therefore, they were considered for the PCA (full data in Supplementary Material).
Table 1 Percentage of the variance accumulated by components 1, 2 and 3 for the selection of the abiotic stress gradient in the rainy season
PC | Eigenvalue | % Variance |
1 | 55073 | 94.348 |
2 | 2559.8 | 4.3853 |
3 | 739.416 | 1.2667 |
Component 1 of the PCA explained 94.35% of the cumulative variance (Table 1). Therefore, it was selected as the abiotic stress gradient for this season and was represented by the abiotic variables potassium (r = 0.10), silt (r = 0.01), clay (r = -0.01), arsenic (r = -0.01), barium (r = 0.12), and cerium (r = 0.03.), cobalt (r = 0.01), chromium (r = 0.03), cesium (r = 0.02), copper (r = 0.01), lithium (r = 0.08), nickel (r = 0.04), lead (r = 0.02), silicon (r = 0.94), strontium (r = 0.02), titanium (r = 0.28), and vanadium (r = 0.03) (Figure 2).
For the dry season, the 44 variables obtained a VIF ≥ 10; therefore, they were considered for the PCA (full data in Supplementary Material). Component 1 explained 97.77% of the cumulative variance (Table 2). Therefore, it was selected as the abiotic stress gradient for this season and was represented by the abiotic variables potassium (r = 0.04), arsenic (r = 0.02), barium (r = 0.11), cerium (r = 0.02), cobalt (r = 0.01), chromium (r = 0.02), cesium (r = 0.02), copper (r = 0.01), lithium (r = 0.07), nickel (r = 0.03), lead (r = 0.01), silicon (r = 0.95), strontium (r = 0.01), titanium (r = 0.26), and vanadium (r = 0.03) (Figure 3).
Table 2 Percentage of the variance accumulated by components 1, 2 and 3 for the selection of the abiotic stress gradient in the dry season
PC | Eigenvalue | % Variance |
1 | 129802 | 97.773 |
2 | 2227.92 | 1.6782 |
3 | 728.39 | 0.54866 |
3.2 Determination and analysis of nematodes in relation to the abiotic stress gradient
For the rainy season, in the CCA (Figure 4), dimension 1 explained 67.73% and dimension 2 explained 18.44% of the data variability, and together, they explained 86.17% of the variance (Table 3); therefore, they were the 2 dimensions considered for the analysis. The CCA showed that there were significant correlations between Globodera and lead and titanium (r = 0.96 and 0.97, respectively); Tylenchus and lead and titanium (r = 0.96 and 0.94, respectively); Helicotylenchus and vanadium (r = 0.99); Trichodorus and cesium (r = 0.84); and Aphelenchus and copper (r = 0.93).
Table 3 Percentage of the variance accumulated by dimensions 1, 2 and 3 for the canonical correspondence analysis in the rainy season
Dimension | Eigenvalue | % |
1 | 0.31032 | 67.73 |
2 | 0.084486 | 18.44 |
3 | 0.06339 | 13.83 |
For the dry season, in the CCA (Figure 5), dimension 1 explained 64.93% and dimension 2 explained 19.98% of the data variability, and together, they explained 84.91% of the variance (Table 4); therefore, they were the 2 dimensions considered for the analysis. The CCA showed that there were significant correlations between Globodera and vanadium, nickel, and titanium (r = 0.87, 0.76 and 0.70, respectively); Tylenchus and vanadium, nickel and titanium (r = 0.91, 0.86, and 0.78, respectively); Meloidogyne and vanadium, nickel and titanium (r = 0.99, 0.98, and 0.95, respectively); Hemicycliophora and lead and strontium (r = 0.91 and 0.88, respectively); and Aphelenchus and Rhabditis and arsenic (r = 0.40 and 0.60, respectively).
Table 4 Percentage of the variance accumulated by dimensions 1, 2 and 3 for the canonical correspondence analysis in the dry season
Dimension | Eigenvalue | % |
1 | 0.54988 | 64.93 |
2 | 0.16922 | 19.98 |
3 | 0.12782 | 15.09 |
In this study, there were both general and specific interactions regarding soil type. Regarding the general interactions, nematodes and abiotic stress gradient variables were indifferent to soil type since the correlations with soil types were not significant, which allowed us to generalize the interactions regardless of the type of soil. Regarding the specific interactions, there were significant correlations in the rainy season between Trichodorus and cerium in the pasture and corn soils and in the dry season between Aphelenchus and Rhabditis and arsenic in the eucalyptus soil. According to Papa et al. (2020) and Petrovskaia et al. (2021), observing both types of interactions allowed us to infer that the number of samples and time periods used were optimal.
In this study, the interactions between the nematodes and the abiotic stress gradient variables had a higher frequency of significant positive correlations (tolerance); in contrast, there were no significant negative correlations (sensitivity). This result agrees with those of Ekschmitt and Korthals (2006), who note that the bioindication of specific soil toxins should preferably be based on tolerant nematodes and not on sensitive ones.
The effects of temporality on the structure and function of a nematode food web can influence the interactions of nematodes and impact variables (da Silva et al., 2021), as observed in this research with the interactions of nematodes and the abiotic stress gradient variables in the rainy and dry seasons; this scenario occurred in this study as these interactions were different, mainly because rainfall is considered one of the environmental variables that most affects the diversity and metabolic activity of nematodes (Song et al., 2017) in that nematodes depend on soil water films to move and capture their food (Nielsen et al., 2014). While fungivorous nematodes are sensitive to disturbances (Sánchez-Moreno & Talavera, 2013), some nematodes of this functional group can be tolerant and therefore indicators of disturbances related to mining (Martinez et al., 2018), as observed in this study with Aphelenchus and copper in the rainy season and arsenic in the dry season. This result was obtained because these nematodes feed on fungi that infect the leaf sheaths and cortex of some roots with concentrations of heavy metals (Seijas, 2004).
Nematodes with survival strategy “r” are resistant to specific disturbances in soil (Gutiérrez et al., 2016), as was observed in this study with Rhabditis and arsenic; thus, this nematode presents a survival strategy “r” and has a high capacity for tolerance to contaminants (Park et al., 2011); having short life cycles (Losi et al., 2021) and being opportunists of soil enrichment (da Silva et al., 2021) and the first colonizers in ecological succession (du Preez et al., 2018), these nematodes are excellent indicators of soil quality in terms of heavy metals (Han et al., 2009).
The presence of organisms of different trophic groups decreases significantly in radioactive soils (Garnier-Laplace et al., 2013); bacterivorous and fungivorous nematodes may be more resistant to soils with this type of element (Lecomte-Pradines et al., 2014). However, in this study, it was observed that Trichodorus, which is an herbivorous nematode, had a high association with the radioactive element cerium, which demonstrates that this type of nematode could present structural and functional characteristics making it tolerant to stressful conditions (Kabir et al., 2018).
Some nematodes may have different tolerance levels toward some heavy metals (Gutiérrez et al., 2016), as in the cases of Tylenchus and Globodera and titanium and lead in the rainy season and Tylenchus, Globodera, and Meloidogyne and nickel, titanium, and vanadium in the dry season. It seems likely that the tolerance of these nematodes to heavy metals provides them the potential for selective adaptation since this capacity is not observed in all herbivorous nematodes (Ekschmitt & Korthals, 2006).
Certain nematodes can be tolerant to soil stressors (Hou et al., 2022), as observed in this research with Helicotylenchus and vanadium in the rainy season, because the exchangeable forms of heavy metals favor the absorption rates of plants that feed on this type of nematode (Park et al., 2011).
4. Conclusions
The bioindicator behavior of the nematodes showed specific interactions between some genera and specific abiotic stress gradient variables; additionally, generalist interactions occurred. However, both in the rainy season and in the dry season, the abiotic stress gradient was highly correlated with bacterivorous, fungivorous, and phytoparasitic nematodes and had a low correlation with omnivorous and predatory nematodes, showing the bioindicator capacity of nematodes for stress parameters that impact soil quality.
In this research, specific and general relationships of nematodes with heavy metals were found, for this reason, the validation of these relationships in other types of soil with mining influence is recommended, this with the purpose of increasing the robustness and effectiveness in the evaluation of soil quality.