1. Introduction
Maize (Zea mays L.) (Poaceae) is the cereal of the American peoples, which has accompanied the development of civilizations; therefore, there is a close association between people and corn agriculture (Serratos, 2009). Capable of adapting to a wide variety of agroecological conditions, maize is the third most important grass in the world after wheat and rice (Sulong et al., 2019; Gupta et al., 2023). By 2020, it was estimated that 201,983,645 ha were harvested worldwide that produced 1,162,352,997 t of maize (FAO, 2022) indicating its global importance in food security. For its part, Latin America produced 209,416,643 t from 38,480,575 ha (FAO, 2022), a region in which maize is destined for human consumption and represents a factor in the survival of indigenous people, peasants and peoples who inhabit it (Serratos, 2009).
Among the limitations of its production, the fall armyworm (FAW), Spodoptera frugiperda Smith (Lepidoptera: Noctuidae), is considered the most relevant pest in maize in several countries in America, Africa, and Asia (Harrison et al., 2022; Nagoshi et al., 2019; Yang et al., 2021; Tay et al., 2023). Based on a review of several investigations, Sun et al. (2021) concluded that S. frugiperda is native to tropical and subtropical regions of the Americas, and their larvae can attack more than 350 plant species, including some representing important crops, such as maize, sorghum, rice, wheat, barley, oats, soybeans, tobacco, tomatoes, cotton, beets, alfalfa, and onions. In addition, they pointed out that S. frugiperda can cause serious yield losses of economically important crops, with maize being the main host plant, where losses range from 15-73%. Among the damage caused to maize, there is the decrease in the photosynthetic area, damage to the whorl structure, direct damage to the grain and the latter directly and negatively affects yield (Chimweta et al., 2020).
According to agro-productive figures from the Agricultural Public Information System of the Ministry of Agriculture and Livestock (MAG) in Ecuador, 435,261 ha were harvested in 2021, in which 1,827,001 t of corn were obtained (MAG, 2022). In this country, the main producing provinces are: Los Ríos, Manabí and Guayas, concentrating 85.03% of the total harvested area (MAG, 2022; Zambrano & Andrade-Arias, 2021). Despite the importance of maize for Latin American and Ecuadorian food sovereignty, several factors interfere with its sustainable production, among which the high use of chemical pesticides stands out (Bolaños & Tapia, 2019).
Based on statistical data from Ecuador's National Institute of Statistics and Census (INEC), a study showed that the use of pesticides and chemical fertilizer was not correlated with the increase in maize productivity, suggesting that the production model has consequences on biodiversity, as well as on other economic and social factors (Bolaños & Tapia, 2019). To guide an integrated pest management program, it is necessary to evaluate alternatives with the least economic, social, and environmental impact. Alternatives include plant resistance, selective use of chemical insecticides, as well as biological insecticides. In this context, the aim of this research was to evaluate alternatives for controlling S. frugiperda in commercial maize hybrids under field conditions at two seasons of the year.
2. Methodology
The research was carried out during two production cycles (June - October 2021 and January - May 2022) in the locality of Jauneche, Palenque canton, Los Ríos province, Ecuador (coordinates: 01°25′58′′S and 79°44′58′′W), located at 31 meters above sea level. The life zone corresponds to a tropical rainforest. The climatic conditions during the production cycles of the maize trials are presented in Table 1.
In each cycle, an experimental lot of maize of approximately 1200 m2 was planted, with three commercial hybrids, which were designed in random blocks evaluating three pesticide treatments as control alternatives. The commercial hybrids used were: ADV-9139®, Trueno NB-7443®, and INIAP-551®. The experimental plots consisted of four rows 5 m long, 0.2 m between plants and 0.8 m in rows, at a population density of 62500 plants ha-1.
To evaluate the control, the treatments applied were: T1: Recommended treatment in the area that consisted of a sequence of insecticides (CLE) based on chlorantraniliprole (100 g L-1 of active ingredient (a.i.)) (dose 200 mL ha-1), followed by lufenuron (50 g L-1 a.i.) (dose 400 mL ha-1 ) and emamectin benzoate (50 g kg-1 a.i.) (dose: 200 g ha-1) applied at 10, 20 and 30 days after sowing (das), respectively. T2: Biological insecticide based on Bacillus thuringiensis subspecies kurstaki (BT) (35 g L-1 a.i.) (dose: 750 g ha-1). T3: Conventional treatment used by farmers in the area based on methomyl (MET) (90 g kg-1) (dose: 200 g ha-1). T4: Untreated plot (UP). Sprays in treatments 2 and 3 were performed at 10, 20, and 30 das.
In each experimental lot, all the agronomic tasks recommended for the normal development of the crop (fertilization, weed control, disease control and irrigation in the summer season) were carried out. Three applications were made for fertilization, the first with complete fertilization (16N-12P-28K-2S; dose: 24 kg ha-1), eight days after sowing (das), while for the second and third fertilization, a mixture of nitrogen and sulfur (N38-7S, dose: 24 kg ha-1) was used and applied at 20 and 35 das. For the con trol of weeds, a pre-emergent herbicide based on a mixture of atrazine (dose: 900 g ha-1) + pendimenthalin (dose: 2 L ha-1) + glyphosate (dose: 2 L ha-1) one day after sowing.
Table 1 Monthly climatic conditions during the evaluation cycles of maize trials
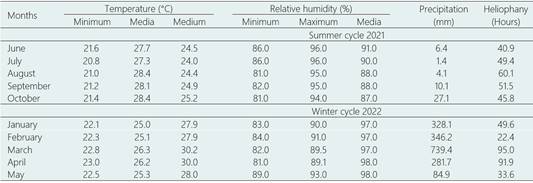
Source: INAMHI, National Institute of Meteorology and Hydrology. Meteorological Yearbooks: 2021 and 2022. Quito, Ecuador.
Post-emergent herbicides, nicosulfuron (dose: 32 g ha-1) and glufosinate (800 mL ha-1) at 15 and 60 days, respectively. Disease control was performed at 35 das using a fungicide based on a mixture of propiconazole + pifeconazole at a dose of 250 mL ha-1. At sixty days after sowing a second application of fungicide using a mixture of azoxystrobin + ciproconazole at a dose of 500 mL ha-1 was performed.
Damage by FAW was evaluated on the buds of the plants and on the cobs. The percentage of plants with the damaged bud (buds damaged with fresh insect droppings) of total plants per plot was estimated. For this, associated with each spray (10, 20 and 30 das) four evaluations were carried out at 0, 3, 6 and 9 days, as well as a final evaluation at 61 das for a total of 13 evaluations in each cycle. The samplings were carried out on the two central rows (50 plants in total) of each treatment by repetition. In the case of cobs, the number of cobs damaged was determined, by counting the total number of cobs, and those affected by worms on all the plants of the two central rows of each treatment.
Plant height and the insertion height of the cob (cm) were also measured and the grain yield per treatment was estimated at 120 days of the crop cycle, harvesting the cobs from the two central rows (50 plants in total). The cobs were shelled and the yield of the dry grain (kg) (13% moisture and 1% impurities) per plot was determined and subsequently, the yield in t ha-1 was estimated.
Economic analysis. Based on the estimated grain yields per ha (t), the increase (in percentage, %) of the grain yield obtained in the plots treated with insecticides with respect to the grain yields of the untreated plots was calculated. Subsequently, the value of the increase in US dollars ($) was estimated with the average prices paid per ton of corn ($327.9 per ton). The cost of the treatment which includes the price of the pesticide plus the costs of the applications of each treatment was calculated. Variable cost represents the cost of treatment plus the cost of transportation and harvesting. Marginal utility is the result of subtracting variable costs from the percentage increase.
Data analysis. An experimental design of random blocks with factorial arrangement 2 (cycles) x 4 (three pesticides and control) x 3 (hybrids), with 4 replications, was used. The data were subjected to an analysis of variance and Tukey's test (p < 0.05) was used for the comparison of means. The relationship between the variables was tested using a Pearson correlation analysis (p < 0.05). A heat map was made to observe the effect of interactions (hybrid, season and pesticide). The analyses were carried out with the R Development Core Team free software (2022).
3. Results and discussion
The analysis of variance showed significant differences in the season factor for the variables plant damage (p < 0.01), cob damage (p < 0.01) and plant height (p < 0.05). Hybrid factors and pesticides showed significant differences in all variables evaluated (Table 2). The interaction of season x hybrids showed significant differences in plant damage and grain yield, while the interaction of season x pesticides showed significance in the variables plant damage and cob damage. The interaction of hybrids x pesticides was significant in the variables plant height and cob insertion height (Table 2).
Damage by FAW in plants and cobs was significantly lower in the hybrid ADV-9139 (Table 3). As for the seasons, the plants were more damaged in the dry season, while the greatest damage to the cob occurred in the rainy season (Table 3). The lowest plant damage was observed in the plots treated with CLE, while the damage to the cobs was lower in the plots sprayed with both BT and CLE (Table 3).
Table 2 Mean squares of the analysis of variance of the variables evaluated in the study of alternatives for the control of fall armyworm in maize hybrids
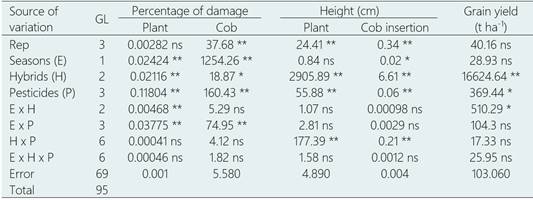
**significant at P < 0.01; *significant at P < 0.05; ns = not significant; GL = degrees of freedom. Percentage of plant damage per plot (Transformed with arcsine) and plant height (cm) (Transformed with square root).
Table 3 Average values of the variables evaluated in the study of alternatives for the control of fall armyworm in maize hybrids
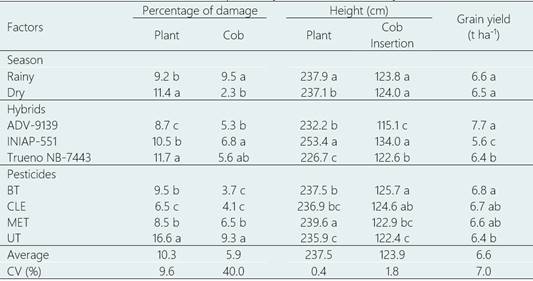
Averages with different letters in a column within the same factor indicate statistical differences according to Tukey's test (P<0.05). BT: Bacillus thuringiensis, CLE: chlorantraniliprole, lufenuron and emamectin, MET: methomyl and UP: untreated plots.
The lower plant and cob damage detected on the ADV-9139 hybrid suggests partial levels of resistance of the hybrid to FAW attack. Plant resistance is a relevant strategy in pest management, and in maize genotypes resistance to FAW damage may be related to physical and chemical factors (Nuambote-Yobila et al., 2023). Chiriboga et al. (2021) report that detecting resistant maize genotypes could be considered a fundamental aspect in the development of sustainable alternatives for the management of this voracious insect.
The greatest damage to plants detected in the dry season coincides with those observed in a study conducted during 2019-2020 in several maize-producing districts of Manica province, Mozambique (Caniço et al., 2020). This study showed that plant damage was higher in the dry season, with percentages ranging from 30 to 66% (Caniço et al., 2020). Likewise, in research carried out by Cokola et al. (2021) in the South Kivu province, Democratic Republic of the Congo, in two agroecological zones, and in the dry and rainy seasons. They detected that plant infestations by S. frugiperda were favored by warm climates, as well as low precipitation, and pointed out that rain seem to limit infestations by FAW. Although the damage to the cob was higher in the rainy season, it was approximately 10% and was lower than the maximum values of 21% of damaged cobs detected in a trial conducted in different locations in tropical and subtropical climates in China (Yang et al., 2021).
Our results contrast with those obtained by Pradeep et al. (2022) who recorded approximately 1.1% cob damage on maize, in a field trial conducted on autumn and winter-spring maize crops in the Karnataka region, India. These authors concluded that the damage caused by FAW on the cob was scarce or insignificant.
The lower damage of plants estimated in the plots treated with CLE as well as the lower damage on the cobs detected in this treatment and in plots treated with BT, suggest the effectiveness of these pesticides to reduce damage to plants and cobs. This is similar and contrasting with what has been obtained in other investigations. A laboratory trial showed the effectiveness of emamectin benzoate and methomyl for the control of S. frugiperda larvae and the low susceptibility of the larvae to spraying with B. thuringiensis var kurstaki (Ahissou et al., 2021). A field study conducted in the Anand region, India, assessed the damage caused by S. frugiperda in plants and cobs (Thumar et al., 2020). The study recorded the least damage when the experimental plots were treated with chlorantraniliprole and emamectin benzoate. Han et al. (2023) carried out research to evaluate the effect of pre-transplant applications of various pesticides against FAW damage in maize. The researchers observed that with chlorantraniliprole sprays the crop could be protected for up to 17 days post-application, sug gesting the effectiveness and economy of the method due to the reduction in pesticide sprays. An investigation tested the susceptibility of laboratory strains of S. frugiperda originally collected on maize and rice in different localities of Argentina when sprayed with local strains and the kurstaki subspecies of B. thuringiensis. The percentage of mortality of FAW of third stage varied from 45 to 83% when sprayed with B. thuringiensis kurstaki (Loto et al., 2019). Baiomy et al. (2023) evaluated the effect of combining B. thuringiensis kurstaki with inorganic salts and with the subspecies, B. thuringiensis aegypti to enhance the mortality of FAW larvae. The study found that both KCl and the subspecies combined with B. thuringiensis kurstaki increased the mortality of FAW larvae by more than 60% seven days after application.
The highest plant heights and cob insertion were detected in the treatments that include the hybrids of INIAP-551 (Table 3; Figure 1). Both variables exhibited a highly significant positive correlation (Figure 2). In turn, the grain yield had a highly significant negative correlation with plant height and cob insertion height (Figure 2).
The highest yields were observed in the plots of the ADV-9139 hybrid treated and untreated with pesticides, whose plants showed the lowest values of height and insertion of the cob (Table 3; Figure 3). The lowest yields were detected in the hybrid INIAP-551 in which the plants showed a significantly higher height and insertion of the cob (Table 3; Figure 1).
Plant height and cob insertion height are positively correlated, so there is a positive correlation between plant height and maize yield (Guamán et al., 2020; Possatto-Junior et al., 2017; Zsubori et al., 2002). However, genetic characteristics are specific to each hybrid and are influenced by environmental and agronomic factors (e.g., plant density, fertilization, pests, and diseases). Thus, plant height, cob insertion height, and the estimated grain yields for the ADV-9139 hybrid are similar to those obtained in other investigations (Mendoza & Flores, 2019; Mendoza et al., 2020). The values of plant height and cob insertion height exceed those obtained by Guamán et al. (2020) for the hybrids Trueno NB-7443 and INIAP-551 and plant height values of the latter hybrid are similar to those estimated by Cañadas et al. (2016).
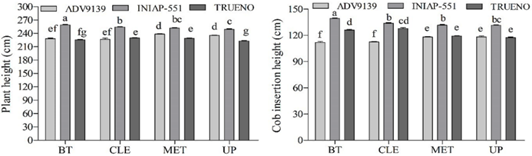
Figure 1 Plant height and cob insertion plant evaluated in the study of alternatives for the control of fall armyworm in maize hybrids. Jauneche, Los Ríos, Ecuador. BT: Bacillus thuringiensis, CLE: chlorantraniliprole, lufenuron and emamectin, MET: methomyl and UT: untreated plots. Averages with the same letter do not differ significantly according to Tukey's test (p<0.05).
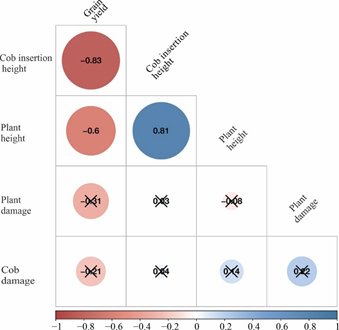
Figure 2 Pearson correlation coefficients (p < 0.05) were computed between the variables evaluated in the study of alternatives for the control of fall armyworm in maize hybrids. Jauneche, Los Ríos, Ecuador. Color intensity and the size of the circle are proportional to the correlation coefficients.
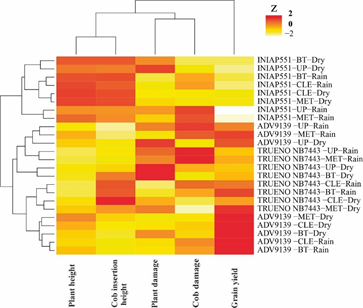
Figure 3 Heat map of the 24 treatments evaluated in the study of alternatives for the control of fall armyworm in maize hybrids. Jauneche, Los Ríos, Ecuador. BT: Bacillus thuringiensis, CLE: chlorantraniliprole, lufenuron and emamectin, MET: methomyl and UP: untreated plots. Color intensity represents the highest values in each variable.
In a heat map, the effect of the seasons, hybrids and treatments with pesticides on the variables of damage, development and grain yield was combined (Figure 3). It was observed that the hybrid Trueno NB-7443 treated with CLE and BT, as well as the hybrid INIAP-551 treated with CLE and BT in the rainy season showed the least damage to the plant. The lower damage to the cob was detected in the hybrid Trueno NB-7443 treated with MET, followed by the hybrid INIAP-551 treated with BT in dry season.
Regarding plant height, the lowest values were estimated on the hybrids Trueno and ADV-9139 in different combinations of treatments, while the lowest cob insertion heights were estimated in untreated plots of the hybrid ADV-9139 in the rainy season. Finally, the highest yields were observed on the treated and untreated plots of the ADV-9139 hybrid in the dry and rainy seasons (Figure 3).
Grain yields on maize plots treated with BT had a yield increase of 2 to 4% higher than in plots treated with the other pesticides evaluated (Table 4). When subtracting variable costs from the increment value, the marginal utility was higher when maize plots were treated with BT, while plots treated with MET showed the second highest marginal utility and plots treated with CLE showed the lowest marginal utility (Table 4). These results differ from those obtained by Divya et al. (2022) who reported greater control coupled with a higher benefit cost when emamectin benzoate and chlorantraniliprole were applied.
Although MET showed the second highest marginal utility after BT for its use, it is necessary to consider that methomyl is moderate to highly toxic to fish and very toxic to aquatic organisms and invertebrates coupled with the fact that it is highly toxic orally to birds and mammals (Van Scoy et al., 2012; Liang et al., 2023). For these reasons, it is important to consider social and environmental costs when estimating crop production costs. Spodoptera frugiperda is a polyphagous pest capable of generating resistance mechanisms to frequent pesticide applications (Kuate et al., 2019; Tay et al. 2023).
Table 4 Economic analysis based on the grain yield and cost of each treatment, and the marginal utility of the maize harvest
Treatments | Grain yield (t ha-1) | Percentage Increase | Increment value ($) | Total Treatment Cost ($) | Variable Cost† | Marginal Utility ($) |
Bacillus thuringiensis | 6.78 | 7% | $141.0 | $66.5 | $81.5 | $59.5 |
Chlorantraniliprole; Lufenuron and Emamectin Benzoate | 6.65 | 5% | $95.0 | $78.14 | $88.3 | $6.7 |
Methomyl | 6.56 | 3% | $69.9 | $29.0 | $36.5 | $33.4 |
Untreated plots | 6.35 | - |
†Variable cost: cost of treatment + cost of transportation and harvesting.
Thus, several alternatives could be implemented for the integrated management of this voracious insect. The resistance of plants, even if partial as shown by the hybrid ADV-9139 can be combined with the use of pesticides with less environmental impact, and in that sense, in this research plots treated with BT showed an increase in grain yield of 7% greater than untreated plots, and in turn, the use of specific insecticides would reduce the collateral effects on the beneficial entomofauna and on the environment in general.
4. Conclusions
In the dry season, there was a higher incidence of plant damage by S. frugiperda, than in the rainy season. While the greatest damage of the cob was found in the rainy season. The ADV-9139 hybrid reported the lowest plant damage in the two periods evaluated and the highest yields. The hybrids INIAP-551 and Trueno NB-7443 showed less plant damage in the rainy season. Treatment with insecticides based on Chlorantraniliprole, Lufenuron, and Emamectin Benzoate (CLE) controls the attack of S. frugiperda, minimizing damage to plants. The product based on B. thuringiensis showed less cob damage, with values similar to the CLE chemical treatment.
In order to reduce the damage in maize caused by the fall armyworm, future studies should focus on evaluating other alternatives with less impact, such as insecticides based on plant extracts, and treatments based on growth-promoting bacteria with biocontrol activity.