1. Introduction
Mashua (Tropaeolum tuberosum) is an Andean tuber that has been cultivated in Peru for centuries due to its significant contribution of proteins (Manrique et al., 2014), carbohydrates, vitamin C, phenolic compounds, and anthocyanins. It has been consumed from ancestral times until today and is attributed to nutraceutical properties related to liver, kidney, and prostate problems. The presence of glucosinolates and phenolic compounds in this tuber has been associated with health problems (Mi˛ Ekus et al., 2020). Additionally, the content of benzylglucosinolate (BGLS) in Mashua plays a protective role against pests and diseases (González-Romero et al., 2021; Leidi et al., 2018).
Significant differences have been found in the content of bioactive compounds and nutritional characterization among some varieties of mashua. However, the nutritional characteristics of yellow, purple, and yellow purple mashua tubers are simi lar, and they are noted to be rich in phosphorus, potassium, and vitamin C. The purple genotype has particularly high values of total anthocyanins, total flavonoids, total phenolics, tannin content, and antioxidant activity (Coloma et al., 2022).
The Glucosinolate content in Mashua presents a variability of 4.9 to 54.2 μmol g-1 of dry matter, with glucoaubrietin being the most predominant compound. In addition, it has been observed that storage and sun exposure have a significant impact on the amount of glucosinolates present in Mashua, since it has been proven that storage in the shade increases its content (Campos et al., 2019).
Glucosinolates are found in different plant families, including Brassicaceae, Capparaceae, and Tropaloeolaceae (Martín & Higuera, 2016). Studies have shown that lactic acid bacteria are capable of biotransforming the glucosinolates of mashua (Tropaeolum tuberosum), using most of the glucosalibin and glucotropaeolin, as well as 46.7% of the glucoaubrietin. These biotransformation processes can generate health-beneficial compounds for consumers (Aguilar-Galvez et al., 2023).
The consumption of foods containing glucosinolates in a diet is important, since the product of glucosinolate hydrolysis, which occurs during preparation and/or chewing, is the isothiocyanates, a compound that promotes cellular apoptosis within the cancerous organism. Glucosinolates are also at tributed with antioxidant, bactericidal and fungicidal properties, as well as being used as bioherbicides and repellents in the field to protect crops. In our country, there are around 300 accessions and so far, there is not enough scientific knowledge about the extraction and quantification of glucosinolates from these tubers, and many of them are in the process of extinction, with a loss of germplasm with an im pressive bioactive potential (Angelino & Jeffery, 2014; Arora et al., 2016).
The new trends in dietary habits and the need for functional foods, with a broadly beneficial effect on health that reduces the risk of various types of diseases, have led researchers around the world to seek crops whose popular use in traditional medi cine can be scientifically proven and industrialized (Aguiló-Aguayo et al., 2014).
The commercialization and consumption of mashua is limited due to the scarcity of scientific studies on its bioactive properties (Alvarez-Jubete et al., 2014). Annual harvesting and inadequate post-harvest handling can decrease the glucosinolate content, and unfavorable organoleptic characteristics can discourage consumption. Homemade preparation is based on convenience and taste preferences, which can affect nutrient and beneficial compound retention. Additionally, there are no studies investigating the effect of processing treatments and conditions on the glucosinolate content in mashua, suggesting the need for processing methods that optimize glucosinolate retention and improve the texture and flavor of the tuber (Alfageme & Rondán, 2022). Aguilar-Galvez et al. (2022) investigated the impact of cold storage and drying process on the glucosinolate composition in mashua-derived products, concluding that the glucosinolate content reached its maximum value after 10 days of cold storage while processing the tubers into flour resulted in significant losses of glucosinolates. On the other hand, Paucar-Menacho et al. evaluated the antioxidant activity and inhibition of carbohydrate digestion of dehydrated mashua and maca powders after in vitro gastrointestinal digestion, concluding that the bioaccessibility and bioactivity of mashua decreased after the steaming process, but the polyphenols from mashua were more effective in modulating carbohydrate digestion than those from maca (Paucar-Menacho et al., 2020).
Mashua has a very high content of glucosinolates (GSL) compared to other plant species (Table 1). For example, the content of 4-hydroxybenzyl in mashua varies between 48.6 and 239.5 µmol/g, while in radish (Raphanus sativus) the content varies between 0.2 and 6.8 µmol/g. Similarly, the content of benzyl, 4-methoxybenzyl, 5-methylsulfinylpentyl, and 4-hydroxy-3-indolylmethyl is considerably higher in mashua compared to other species.
Table 1 Glucosinolate content in different species
Species | 4-hydroxybenzyl (glucosinalbin) | Benzyl (glucotropaeolin) | 4-methoxybenzyl (glucoaubrietin) | 5-methylsulfinylpentyl (glucoalyssin) | 4-hydroxy-3-indolylmethyl (4hydroxyglucobrassicin) |
Mashua (Tropaeolum tuberosum) | 48.6 - 239.5 | 12.8 - 183.4 | 9.6 - 163.3 | 6.7 - 190.4 | 2.1 - 20.8 |
Radish (Raphanus sativus) | 0.2 - 6.8 | 0.2 - 4.7 | 0.2 - 8.3 | 0.1 - 9.9 | 0.1 - 1.4 |
Broccoli (Brassica oleracea var. italica) | 0.2 - 19.2 | 0.2 - 12.4 | 0.3 - 3.4 | 0.1 - 8.8 | 0.1 - 0.6 |
Cabbage (Brassica oleracea var. capitata) | 0.2 - 30.5 | 0.2 - 23.5 | 0.1 - 11.5 | 0.1 - 3.3 | 0.1 - 0.5 |
These values are a compilation of different studies, below I present three authors whose work could be relevant to update the bibliography: (Rosero-Moreano, 2020.; Vásquez-Quezada, 2021).
It is important to note that these values may vary depending on factors such as the variety, growing region, and analysis method used. Additionally, GSL content is not the only factor that determines the nutritional value of a plant, as other components such as vitamins, minerals, proteins, and fiber are also important.
Given the relevance of the topic, the objective of the study was to establish how postharvest storage types (sun, shade, and refrigeration) affect secondary components (glucosinolates).
2. Methodology
2.1 Vegetal material
Purple mashua (Tropaeolum tuberosum), from the province of Yunguyo in the department of Puno. The study sample consisted of 10 kg of purple mashua, to which 20 different treatments were applied: blanching, microwave, dehydration and freeze-drying.
2.2 Evaluation of the influence of bleaching and dehydration treatments of purple mashua
The influence of bleaching and freeze-drying treatments at the time of cutting and after bleaching and freeze-drying treatments was evaluated in relation to myrosinase activity. Each blanched sample was divided into two subgroups, one of which was ground after freezing with liquid nitrogen and stored at -20 °C in high-density polyethylene bags, tightly closed at the time of analysis, while the other was dried as detailed in the following section. All treatments were performed in duplicate.
Moisture, dry matter and myrosinase activity were determined in the processed samples. The experimental design is shown in Figure 1.
2.3 Moisture and dry material determination
The percent gravimetric method Method 920.151 (AOAC INTERNATIONAL, 2019) was used, in which a determined weight of the sample is dried in an oven under vacuum pressure until a constant weight is obtained. The moisture content was calculated by the difference of weights and the dry material was calculated by the difference of the percentage of moisture with respect to 100 percent.
2.4 Determination of glucosinolate content by HPLC
The method described by Grosser & van Dam (2017) was used:
Preparation of solutions required for glucosinolate extraction. 500 mL of 70% methanol (MeOH) in water (ultrapure) was prepared in a glass bottle.
20 mM sodium acetate (NaOAc) (pH = 5.5) was prepared by adding 0.82 g NaOAc or 1.36 g NaOAc x 3 H2O in 500 mL water; the pH was adjusted with hydrogen chloride (HCl). The NaOAc was then stored in the refrigerator.
The column material was then prepared by mixing 10 g of cross-linked dextran gel (type G-25) with 125 ml of ultrapure water, and the resulting mixture was stored in a refrigerator (4 °C) (Ares et al., 2015; Cristóbal et al., 2011;Grosser & van Dam, 2017).
2.5 Preparation of the sulfatase solution
10,000 units of arylsulfatase (type H-1 from Helix pomatia) was dissolved in 30 ml of ultrapure water and 30 ml of absolute ethanol (EtOH) was added. The solution was mixed and transferred to a 250 ml centrifuge tube or several smaller tubes, depending on availability.
The mixture was centrifuged at 2,650 xg for 20 min at room temperature (RT). The supernatant (s) was then transferred to a beaker; 90 ml EtOH was added and mixed. Next, the mixture was centrifuged in one or more centrifuge tubes at 1,030 xg for 15 min at room temperature.
The supernatants were then discarded. Next, the pellets were dissolved and combined in a total of 25 ml of ultrapure water. It was then vortexed well, dispensed into 1 ml tubes and stored in a freezer at -20 °C.
2.6 Preparation of the sinigrin reference solution
About nine mg of sinigrin monohydrate was weighed to the nearest 1 µg (e.g., 8769 mg). then transferred to a 10 ml volumetric flask and sinigrin monohydrate was dissolved in 10 ml of ultrapure water. The molarity of the stock solution was calculated and five sinigrin references between 50-750 µM were prepared by diluting the stock solution. The different sinigrin references were dispensed into 1.5 ml reaction tubes and frozen at 20 °C; then, a series of five sinigrin references were included in each extraction batch. The correct molarity values for the currently used sinigrin references were used when calculating the concentrations.
2.7 Extraction of glucosinolates
The process followed consisted of:
Freeze-dried, finely ground vegetal material (generally 50 - 100 mg dry weight; final glucosinolate concentrations in the extract should be in the range of the reference curve) was weighed to the nearest 0.1 mg in 2 ml, labeled, round-bottom reaction tubes. Two small metal balls (3 mm diameter) were added as boiling retardants to each tube.
For fresh samples, they were flash frozen and then ground under liquid nitrogen freezing and kept frozen until extraction. For this, the amount of material weighed for extraction and the percentage of MeOH in the extraction liquid was increased to 85% to compensate for dilution by water in the materials.
1 ml of 70% methyl alcohol (MeOH) was pipetted into each tube and vortexed briefly. The tubes were closed with safety caps before being placed, as quickly as possible, in a hot water bath (90 - 92 °C) for a few minutes (~ 5 min), until the 70% MeOH just boiled off.
The sample tubes were placed in an ultrasonic bath for 15 min. In the meantime, the sulfatase and the five sinigrin reference samples were removed from the freezer to thaw at room temperature.
After ultrasonication, the sample tubes were centrifuged at 2700 xg in a benchtop centrifuge for 10 min at room temperature; a pellet should form in each tube. Next, the supernatants were added to the labeled columns and the five reference samples were pipetted, in separate columns.
While pipetting the supernatants, the tip is held over the pellet to avoid pipetting the vegetal materials. It was kept in mind that when using dried samples, the volume of the supernatant will be less than 1.0 ml. 1 ml of 70% MeOH was added to the remaining pellets in the sample tubes and the tubes were shaken before being placed in an ultrasonic bath for 15 min. The tubes were centrifuged again, as in the previous step, and the supernatants were added to the respective columns; due to the properties of the column material, the negatively charged sulfate group of the glucosinolates was specifically retained on the column.
The columns were washed with the extracts in three sequential steps.
a. 2 x 1 ml of 70% MeOH was pipetted into each column and waited for the column to dry before adding the next 1 ml; this removed more apolar compounds from the extracts (e.g., chlorophyll).
b. The MeOH was rinsed by adding 1 ml of ultrapure water to each column.
c. 2 x 1 ml of 20 mM NaOAc buffer was pipetted into each column to create optimal conditions for the sulfatase reaction. The rack with the columns was removed from the waste tray and the rack feet were wiped dry with a tissue. Next, the rack was placed on top of the block with labeled vials and tubes, making sure that each column tip was in the corresponding labeled 2 ml tube. We added 20 µl of sulfatase solution to the columns and made sure that the sulfatase reached the sur face of the column material. Next, 50 µl of NaOAc buffer was pipetted into each column to remove the sulfatase, and then the columns were covered with aluminum foil and allowed to stand overnight, which will, due to sulfatase activities, remove the sulfate group, releasing the desulfoglucosinolates from the column so that they can elute with water. The next day, elute the desulfoglucosinolates by pipetting 2 x 0.75 ml of ultrapure water into each column. When all columns were dry, the column support was lifted and removed from the reaction tubes.
d. The tubes were capped (make sure there are holes in the caps) and frozen in liquid nitrogen or in a freezer at -80 °C for 30 minutes. The samples were then frozen for 12 - 24 h (depending on the number of samples and the capacity of the freeze dryer) to remove all water.
After lyophilization, the residue was redissolved in an exact volume (usually 1.0 ml) of ultrapure water, and the samples and the five Sinigrin references were transferred to labeled HPLC vials. The samples were kept in a refrigerator (4 °C) for up to two weeks or alternatively in a freezer (-20 °C) for up to one year before HPLC analysis.
The glass columns were left to dry under the hood overnight and discarded when dry. The metal beads were then retrieved from the sample tubes used for reuse.
2.8 HPLC measurements of extracted samples
Glucosinolates were separated on a reversed-phase C 18 column (4.6 x 150 mm, 3 µm, 300 Å) with an acetonitrile-water gradient and a flow rate of 0.75 ml/min and a column temperature of 40 °C. Detection and quantification were then performed at 229 nm (Collett et al., 2014).
Sinigrin references were measured on the same column, but with a gradient method adapted to reduce the use of solvents. Next, the five Sinigrin references were injected, starting with the reference with the lowest concentration. The samples were then injected, including an injection of methanol (blank) after every tenth sample, to clean the column and avoid peak carryover.
2.9 Identification and quantification of glucosinolates
A. Identification
UV spectra and retention times of peaks in the samples were compared with commercially available pure glucosinolate references or literature references.
B. Quantification
The area under the peaks of the five sinigrin references and the samples were integrated.
A calibration curve of the five sinigrin references measured as a function of the integrated area was then calculated. The regression line equation (Equation 1) was used to calculate the interpolated amount of glucosinolates (Equation 2).
Next, to calculate the concentration of the differrent glucosinolates, the interpolated amount (x) was multiplied by the response factor (M) for de tection at 229 nm. Consensus response factors of the most detected desulfoglucosin olates (Table 4).
Then, to calculate the final amount of glucosinolates (x t) in the sample, the dilution factor (D) and the sample mass (w) used for the analysis are considered (Equation 3).
Where y: peak area; m: slope of the 5-point benchmark regression curve; c = the intersection of the regression line with the y-axis; x = amount of glucosinolates in the extract; x t = concentration of glucosinolates in the plant sample; D: dilution factor; M: response factor for detection at 229 nm of Buchner; w: mass of the sample used for extraction.
Formulas, data processing and analysis used for quantification:
a. Glucosinolate content in micromoles per gram of dry tissue (µmol/g dry weight) (Eq. 1).
Where [GSL]: Glucosinolate concentration (µmol/ g dry weight); A: HPLC area; Wmta: Weight of sample used to prepare the extract (g); Vext: Volume of extract (ml); Vcol: Volume of extract added to the column (ml); Vf: Final volume (ml); FR: Response factor; DM: Dry matter content; PM: Molecular weight of glucosinolate (g/mol); Fd: Dilution Factor.
b. Myrosinase activity (Eq. 2). Calculation of remaining myrosinase activity in nanomoles of transformed Sinigrin per gram of dry tissue base per minute (nmol/g min dry weight), by measuring substrate consumed.
Where MYR: Myrosinase activity (nmol/g dry weight min); V Bl : HPLC area of the blank; A Trat : Treatment HPLC area; Wmta: Weight of sample used to prepare the extract (g); Vext: Volume of the enzymatic extract (ml); Vcol: Volume added to the column (ml); V fBl : Final volume of the blank (ml); V fTrat : Final Volume of Treatment (ml); MS: Dry Matter Content; PM: Molecular weight (g/mol); t Rx : Enzymatic Reaction Time (min); Fd: Dilution Factor.
c. Statistical analysis
The results are presented in tables and graphs and subjected to an Analysis of Variance and Duncan and Tukey's Test to test the differences between means, at 95% confidence, for which statistical software Statgraphics Centurion XV version 15.2 (StatPoint Inc., Warrenton, Virginia, USA) and statistical program INFO STAT free version 2018 were used.
3. Results and discussion
Quantification of glucosinolates in fresh purple mashua
The fresh purple mashua (control) was analyzed for its profile and content of glucosinolates (Figure 2); according to their class they are identified as one aliphatic, one indolic, and three aromatics. It is worth noting that this species is very characteristic of these secondary metabolites.
These glucosinolates correspond to the identification of five glucosinolates in fresh purple mashua, three of aromatic nature, one aliphatic and one indolic. The aromatic glucosinolates identified were 4-hydroxybenzyl (glucosinalbin), benzyl (glucotro-paeolin), and 4-methoxybenzyl (glucoaubrietin), while the aliphatic glucosinolate identified was 5-methylsulfinylpentyl (glucoalyssin) and the indolic was 4-hydroxy-3-indolylmethyl (4hydroxygluco-brassicin) (Table 2). In addition, it is mentioned that (Fahey et al., 2001; Ortega et al., 2006) reported the presence of two different types of glucosinolates in the Tropaeolaceae family, which are aliphatic derivatives of methionine, isoleucine and valine, and aromatic derivatives of phenylalanine and tyrosine. However, the authors are not mistaken in stating that the precursors of glucosinolates in mashua are phenylalanine and tyrosine. According to the article by Fahey et al., published in the journal Phytochemistry in 2001 (Fahey et al., 2001), glucosinolates (GLS) are a class of secondary compounds that contain sulfur and nitrogen, and that are derived from amino acids. The GLS present in mashua correspond to: 4-methoxybenzyl glucosinolate (glucoaubrietin) which represents 96-99 % of total GLS (tGLS), followed by benzyl glucosinolate (glucotropaeolin) and two isomers of 4-hydroxybenzyl glucosinolate (glucosinalbin) (Campos et al., 2019; Valcárcel-Yamani et al., 2013). These GLS are formed from the condensation of an amino group with a sulfate group activated by ATP, followed by the addition of a glucose unit. The amino acid precursors can be modified by various enzymatic processes that give rise to the structural diversity of GLS. Phenylalanine and tyrosine are the precursors of aromatic GLS, such as those found in mashua (Fahey et al., 2001), In addition, Aguilar-Galvez et al. (2023) identified and corroborated the presence of five glucosinolates in fresh purple mashua using high-performance liquid chromatography with UV detection and tandem mass spectrometry, providing and confirming their names, highlighting the importance of glucosinolates as characteristic secondary metabolites of this species.
Quantification of glucosinolates in processed purple mashua
The results of the glucosinolate content of mashua stored under refrigeration, shade, and exposure to sunlight are shown in Table 2. The statistical analysis showed that there is a significant difference (p < 0.05) in the total glucosinolate/glucoaubrietin content and type of storage: shade content is higher, followed by refrigeration content, and the least in those exposed to sunlight; however, for the other glucosinolates, there is no significant difference between refrigeration and shade storage, but with storage exposed to sunlight, the latter is present in smaller amounts, at a maximum of five for the formulated items. Regarding the indicator "motivation towards saving," the following was found: an average of 2.19, a mode of 1.25, a minimum value of 1, and a maximum value of 5 for the formulated items.
Different studies have evaluated the glucosinolate content in mashua and its variation during storage under different conditions. Malpartida et al. (2022) analyzed the variation of glucosinolates in mashua during tuber development, as well as the effect of different cooking methods. Campos et al. (2022) studied the composition of glucosinolates and phenolic compounds in mashua and how postharvest cooking affected the stability of glucosinolates and myrosinase activity in Andean mashua tubers (Tropaeolum tuberosum), finding changes in glucosinolate content and antioxidant capacity during mashua storage . Additionally, they evaluated the effect of storage temperature on glucosinolate content in mashua tubers and found a decrease in its content after 30 days at 15°C (Aguilar-Galvez et al., 2023; Marín-Tello et al., 2021). Other studies have evaluated the influence of different processing methods on glucosinolate content in mashua, such as blanching and drying.
Table 3 shows the total glucosinolate content of purple mashua flour after undergoing 20 different blanching and drying treatments. In the case of fresh tubers, that is, without microwave blanching with samples exposed to sunlight, significant reductions in total glucosinolate content ranging from 32.96% to 90.67% (p > 0.05) were observed, while boiling blanching resulted in non-significant increases of 0.05% (p > 0.05) and significant reductions of up to 39.42% (p > 0.05), depending on the treatment.
For fresh tubers, i.e., without microwave bleaching with sun-exposed samples, significant reductions in total glucosinolate content of 32.96 to 90.67% (p > 0.05) were generated, while boiling bleaching generated non-significant increases of 0.05% (p > 0.05) and significant reductions of up to 39.42% (p > 0.05), depending on the treatment. For sun-exposed tubers, microwave blanching generated significant reductions in total glucosi-nolate content from 49.65% to 84.11% (p > 0.05); while boiling blanching generated non-significant reductions from 7.99% (p > 0.05) to significant reductions up to 32.37% (p > 0.05), depending on the treatment.
Drying at 60 °C generated from non-significant increases by 2.21% (p > 0.05) to significant reductions up to 90.67% (p > 0.05), while drying at 80 °C was generated from non-significant increases. Significant increases from 0.05% (p > 0.05) to significant reductions up to 88.15% (p > 0.05), depending on the treatment.
The highest values of total glucosinolate content were obtained for tubers without sun exposure, without blanching and dried directly at 60 °C (113.04 µmol g-1 dry weight); and, while tubers without sun expo sure, cut, boiled, blanched and dried at 80 °C (110.66 µmol g-1 dry weight), showed statistically similar values (p > 0.05) to the total glucosinolate content of fresh unprocessed mashua (Arias, 2011).
Different studies have evaluated the effect of blanching and drying on the glucosinolate content in mashua. However, direct exposure to sunlight for 15 days substantially reduced the glucosinolate content and showed an 86% greater loss compared to refrigerated and shaded storage (Campos et al., 2019). In addition, Celis (2020) evaluated the effect of drying temperature on the glucosinolate content and antioxidant capacity of mashua flour.
Table 3 Multiple range test, Duncan's method to evaluate the glucoalyssin content in freeze-dried mashua flour
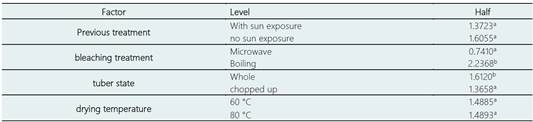
The lowest values of total glucosinolate content were obtained for tubers without sun exposure, cut, microwave bleached and dried at 60 °C (10.32 µmol g-1 dry weight) and at 80 °C (13.10 µmol g-1 dry weight) with a significant reduction of 90.67% and 88.15% respectively (p > 0.05).
Glucoalyssin content
Glucoalyssin content, the only aliphatic glucosinolate present in the purple variety, was significantly affected by blanching treatment and tuber condition (p > 0.05). Pretreatment and drying temperature did not have a significant effect, but there were interactions between factors (p > 0.05. Also, using the multiple range test and Duncan's multiple comparison procedure, statistically significant differences were determined between the levels of the same factor for the four factors evaluated (Table 3).
Given the findings presented, the contribution of each factor was measured disregarding the effects of the other factors, it was necessary to consider the differences between each treatment. Table 4 shows the glucoalyssin content of mashua flour, purple variety, after being subjected to 20 different bleaching and drying treatments.
For fresh tubers, i.e., without sun exposure, microwave bleaching generated significant reductions in glucoalyssin content from 66.05% to 94.99% (p > 0.05); while boiling bleaching generated significant reductions from 47.76% (p > 0.05) to 69.27% (p > 0.05), depending on the treatment.
For sun-exposed tubers, microwave blanching generated significant reductions in glucoalyssin content from 60.34% to 86.50% (p > 0.05); while boiling blanching generated significant reductions from 33.44% (p > 0.05) to significant reductions of up to 51.63% (p > 0.05), depending on the treatment.
Drying at 60 °C generated from significant reductions from 33.44% to 94.99% (p > 0.05), while drying at 80 °C generated significant reductions from 39.36% (p > 0.05) to 93, 22 % (p > 0.05), depending on the treatment.
The highest values of glucoalyssin content were obtained for tubers without sun, without blanching and dried directly at 60 °C (2.57 umol. g-1 DRY WEIGHT), and in tubers exposed to the sun, whole, blanched and dried in boiling at 60 °C (2.63 umol. g-1 dry weight), with a significant decrease of 44.03% and 33.44% (p> 0.05), respectively, compared to the content of glu cose analysis of fresh, unprocessed mashua (Chan, 2015).
Different studies have evaluated the effect of drying on the glucoalyssin content in mashua (Celis, 2020) evaluated the effect of blanching and drying on the glucoalyssin content and antioxidant capacity of mashua. Additionally, other studies have evaluated the influence of different processing methods on glucosinolate content in mashua, such as blanching and drying (Coronado & Castillo, 2013).
The lowest values of glucoalyssin content were obtained in tubers without sun exposure, cut, microwave blanched and dried at 60 °C (0.23 µmol g-1 dry weight) and at 80 °C (0.31 µmol g-1 DRY WEIGHT), with a significant decrease of 94.99% and 93.22%, respectively (p > 0.05) (Table 4).
4-Hydroxyglucobrassicin Content
The content of 4-hydroxyglucobrassicin, the only indole glucosinolate present in the purple variety, was significantly affected by pretreatment, blanching treatment and tuber condition (p > 0.05). Drying temperature did not have a significant effect, but it did in some of the interactions between factors (p > 0.05).
The contribution of each factor was measured without considering the effects of other factors, it was necessary to consider the differences between each treatment (Table 5). For fresh tubers, i.e., without sun exposure, micro wave blanching generated non-significant 4-hy droxyglucobasicin reductions of 17.95% (p > 0.05) to significant reductions in content of 93.84% (p > 0.05), while boiling bleaching generated significant increases in 4-hydroxyglucobasicin content up to 24.55% (p > 0.05) and significant reductions up to 25.86% (p > 0.05), depending on the treatment. For sun-exposed tubers, microwave blanching generated significant reductions in 4-hydroxyglucobasicin content from 46.05% to 88.32% (p > 0.05), while boiling blanching generated significant increases up to 21.02% and non-significant reductions up to 19.85% (p > 0.05), depending on the treatment.
Drying at 60 °C generated significant increases up to 24.94% and significant reductions up to 93.84% (p > 0.05) while drying at 80 °C generated significant increases up to 24.5% and reductions up to 89.89% (p > 0.05), depending on treatment.
The highest levels of 4-hydroxyglucobrassicin were obtained in tubers without sun exposure, without bleaching and dried directly at 60 °C (0.16 µmol g-1 dry weight), and in tubers without sun exposure, whole, boiling blanched and dried at 80 °C (0.16 µmol g-1 dry weight), a significant increase of 24.94% and 24.55% (p > 0.05), respectively, compared to the 4-hydroxyglucobrassicin content in fresh unprocessed mashua.
The lowest values of 4-hydroxyglucobrassicin content were obtained for tubers without sun exposure, cut, microwave blanched and dried at 60 °C (0.008 µmol g-1 dry weight) and at 80 °C (0.013 µmol g-1 dry weight), with a significant reduction of 93.84% and 89.89%, respectively (p > 0.05).
Glucosinalbin content
The content of glucosinalbin, one of the three aromatic glucosinolates present in the purple variety, was significantly affected, only by bleaching treatment (p > 0.05). Pretreatment, tuber condition and drying temperature had no significant effect but did in some of the interactions between factors (p > 0.05) (Table 6). For fresh tubers, i.e., without sun exposure, microwave bleaching generated non-significant reductions of 40.37% (p > 0.05) to significant reductions of up to 99.22% (p > 0.05) in glucosinalbin content; while boiling bleaching generated non-significant reductions of 19.16% (p > 0.05) to significant reductions of up to 58.10% (p > 0.05), depending on the treatment.
Table 5 4-Hydroxyglucobrassicin content (µmol/g dry weight) for mashua (Tropaeolum tuberosum) purple variety
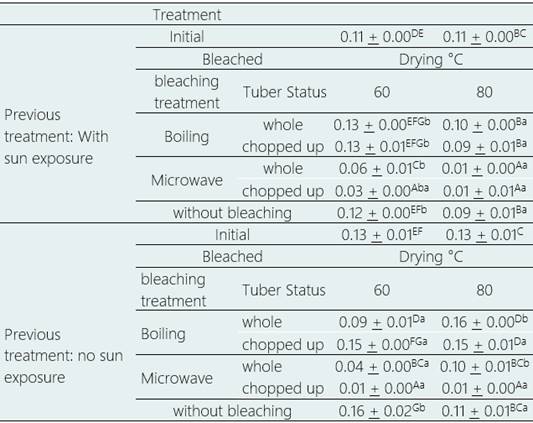
For sun-exposed tubers, microwave blanching generated non-significant reductions of 2.16% (p > 0.05) to significant reductions of up to 80.16% (p > 0.05), while boiling blanching generated significant increases of up to 38.94% (p > 0.05) and non-significant reductions of up to 36.57% (p > 0.05), depending on the treatment.
Drying at 60 °C generated non-significant increases by 5.89% to significant reductions of up to 99.22% (p > 0.05), while drying at 80 °C generated non-significant reductions of 19.16% (p > 0.05) to significant reductions of 96.66% (p > 0.05), depending on the treatment.
The highest values of glucosinalbin content were obtained in tubers without sun exposure, without bleaching and dried directly at 60°C (0.16 umol. g-1 dry weight), and for sun-exposed tubers, whole, boiling blanched and dried at 60 °C (0.13 µmol g-1 dry weight), a non-significant increase of 5.88% and a significant increase of 38.94% (p > 0.05), respectively, compared to the glucosinalbin content in fresh purple Mashua, without processing.
The lowest glucosinalbin content was reached in tubers without sun exposure, cut, microwave blanched and dried at 60 °C (1.2 E-03 µmol g-1 DRY WEIGHT) and 80 °C (4.9 E-03 µmol g-1 DRY WEIGHT), with a significant decrease of 99.22% and 96.66% (p > 0.05), respectively.
Glucotropaeolin content
All treatments led to the loss or total disappearance of glucotropaeolin, one of the three aromatic glucosinolates present (although in low amounts) in the purple variety of fresh mashua.
Glucoaubrietin content
There is a statistically significant difference between the treatments of mashua flour, purple variety, after having been subjected to 20 different bleaching and drying treatments, at the time of bleaching and the bleaching treatments (p > 0.05), being the tubers without sun exposure, the whole tubers and those bleached by boiling, respectively, where the highest content of glucoaubrietin is recorded.
With respect to drying temperature, there is no statistically significant difference between drying at 60 °C and drying at 80 °C (p > 0.05) (Table 7).
The contribution of each factor was measured neglecting the effects of the other factors, it was necessary to consider the differences between each treatment. For fresh tubers, i.e., without microwave blanching, there were significant reductions in glucoaubrietin content of 31.37% up to 90.44% (p > 0.05); whereas boiling blanching, generated non-significant increases of 2.44% (p >0.05) and significant reductions of up to 37.98% (p > 0.05), depending on the treatment.
For sun-exposed tubers, microwave blanching generated significant reductions in glucoaubrietin content from 49.03% to 83.98% (p > 0.05), while boiling blanching generated non-significant reductions from 6.68% (p > 0.05) to significant reductions up to 31.89% (p > 0.05), depending on treatment.
Drying at 60 °C generated non-significant increases of 4.42% (p > 0.05) to significant reductions of 90.44% (p > 0.05) while drying at 80 °C generated non-significant increases of 2.44% (p > 0.05) to significant reductions of 87.90% (p > 0.05), depending on the treatment.
The highest values of glucoaubrietin content were obtained for tubers without sun exposure, without blanching and dried directly at 60 °C (110.16 µmol g-1 dry weight); and for tubers without sun exposure, cut, blanched by boiling and dried at 80 °C (108.06 µmol g-1 dry weight), being these values statistically equal (p > 0.05) to the value of glucoaubrietin in purple mashua (Clarke, 2010).
Different studies have evaluated the effect of blanching and drying on the glucoaubrietin content in mashua. Celis (2020) evaluated the effect of blanching and drying on the glucoaubrietin content and antioxidant capacity of mashua. Additionally, other studies have evaluated the influence of different processing methods on glucosinolate content in mashua, such as blanching and drying.
The lowest values of glucoaubrietin content were obtained in tubers without sun exposure, cut, microwave blanched and dried at 60 °C (10.08 µmol g-1 dry weight) and 80 °C (12.77 µmol g-1 dry weight), with a significant reduction of 90.44% and 87.89%, respectively (p > 0.05).
Myrosinase activity of purple mashua samples
The effect of bleaching (enzyme inactivation) was evaluated by three factors: pretreatment (tubers exposed to the sun and tubers without sun exposure), bleaching treatment (boiling and microwave), and tuber condition at the time of bleaching (whole and sliced).
Myrosinase activity in processed purple mashua was significantly affected by pretreatment (p > 0.05), blanching treatment (p > 0.05), tuber condition at blanching (p > 0.05) and the interaction between these three factors (p > 0.05).
Myrosinase from boiling bleached mashua showed, for sun-exposed tubers, an abrupt and significant 94.8% decrease in activity when the tuber entered the whole bleaching treatment; and, a total loss of activity, when the tuber entered sliced compared to fresh, unbleached mashua (p > 0.05); for tubers without sun exposure, it was observed that there were significant abrupt reductions of 88% and 90.9% for whole and sliced tubers, respectively (p > 0.05). In the case of microwave-bleached mashua, for both whole and sliced tubers, exposed to the sun or without sun exposure, myrosinase showed a total loss of its catalytic activity (Avato & Argentieri, 2015; Becker et al., 2015; Ghawi et al., 2011).
4. Conclusions
The concentration of glucosinolates in mashua, purple variety, is significantly affected by the pretreatment of sun exposure, bleaching, tuber condition and the interactions among them, but not by the freeze-drying process.
Myrosinase activity is significantly affected by sun pretreatment and bleaching, so glucosinolate concentrations and residual myrosinase activity depend on the method and duration of the processing, as well as the interaction between them.
Higher values for total glucosinolate content were obtained for tubers without sunning, unbleached and dehydrated directly at 60 °C (113.04 µmol g-1 dry weight) and for tubers exposed to sunning treatments, cut, blanched by boiling at 80 °C and then freeze-dried (110.66 µmol g-1 dry weight), these values being similar to the total value of glucosinolate content in fresh raw mashua (p > 0.05).
The lowest total glucosinolate contents were obtained for tubers without sun exposure, cut, microwaved, and dehydrated at 60 °C (10.32 µmol g-1 dry weight) and 80 °C (13.10 µmol g-1 dry weight), with a significant reduction of 91 and 88%, respectively (p> 0.05).
Finally, blanching, drying, and freeze-drying methods minimize nutrient losses and provide better physical quality.