1. Introduction
Cocoa production is affected by several diseases, including Moniliophthora roreri, the causal agent of frost rot of the pod, which is the most aggressive disease in the field, as well as 'witches broom,' whose causal agent is Moniliophthora perniciosa and black pod, caused by Phytophthora spp. (Bailey & Meinhardt, 2016) also demonstrated the finding of a viable spore of the phytopathogen in 1-month-old young plants (Phillips-Mora & Wilkinson, 2007). Once the spores are on the surface of the young fruit, they begin to germinate when it is found with ideal favorable conditions, starting the colonization of the fruit through specialized structures growing through the stomata, growing between the cells of the cortex, which generates the production of conidia inside and on the surface of the fruits. The presence of necrosis, deformation, and rot of the ear differentiates the external damage of the fruit. However, some fruits of 60 and 80 days can finish their development without presenting symptoms of the disease in the outer part but can initiate damage to the internal tissue, causing the loss of the fruit (Bailey et al., 2018).
The biological control of M. roreri is a viable alternative. It is increasingly accepted by small, medium, and large farmers to reduce the inoculum present in the field due to the use of beneficial microorganisms, which have antagonistic capacity on phytopathogens through different mechanisms of action and with a broad spectrum (Leiva et al., 2020; Serrano et al., 2021). The species of the genus Trichoderma have been characterized by their overall potential in agriculture, generally used for biological control against various phytopathogens, including M. roreri (Galarza et al., 2015).
Recently, beneficial microorganisms subjected to an encapsulation or microencapsulation process have gained strength due to the protection microorga-nisms give to resist certain adverse conditions and regulate their release (Mancera-López et al., 2019).
Beneficial microorganism formulations include biopolymers such as alginate, agar, starch, carrageenan, dextrin, sucrose, carboxymethylcellulose, and chitosan, among others, into a process including a mix of the ingredients with the polymer solution (Rojas-Sánchez et al., 2022). In this sense, we proceeded with the microencapsulation of formulations of the genus Trichoderma using alginate and biopolymers as a base, preserving their condition for up to 14 months in storage (Locatelli et al., 2018). The encapsulation process protects the inoculum of the beneficial fungus against UV rays and ensures long-term viability (Mancera-López et al., 2019).
Additionally, the strains that go through the encapsulation process keep the chitinolytic and cellulosic properties of the Trichoderma strains used for biological control (Maruyama et al. 2020). Therefore, the use of alternative methods reduces the application of pesticides or chemical fertilizers while maintaining high effectiveness and performance standards. Microcapsules have, also, become popular due to their technically simple operation and short preparation times, which also maintain the viability of the encapsulated microorganisms (Martinez et al., 2023; Qi et al., 2023). Despite all these favorable points in the production and application of microcapsules, there is limited information regarding the different materials for the creation of the encapsulates and even their potential effectiveness in several crops of commercial interest, which is what motivates us to continue performing studies based on new biocontrol alternatives.
The objective of this study was to obtain microcap sules from a Trichoderma spore solution using so dium alginate and calcium acetate as matrix and its subsequent use both under in vitro and in vivo conditions for the biological control of M. roreri in commercial cocoa plantations in Guayas province Ecuador.
2. Methodology
Fungal strains
The fungal strains used in this study belong to the Microorganisms Collection of the Biotechnological Research Center of Ecuador of the Escuela Superior Politécnica del Litoral (CIBE-ESPOL), which were isolated from cocoa plantations in the Canton of Naranjal Province of Guayas. The beneficial strains selected were T. ghanense (code C4B) and T. reesei (code C2A) and the pathogenic strain M. roreri (code MR59). The strains were reactivated, reseeded three times, and incubated at 28 °C for seven days for the strains to recover their viability.
Mass microencapsulation of Trichoderma spp.
The test began with the calibration of the microencapsulation system; three different tests were prepared with the strains of T. ghanense and T. reesei, where the variables to be analyzed were height, speed, and time of spraying, which are known to affect the size and shape of the microcapsules (Table 1). Then, 500 µl of the T. ghanense and T. reesei strains were added in 50 ml of 1.5% sodium alginate, and the resulting solution was sprayed on the tank containing a volume of 5 L of 2% calcium acetate, keeping it under constant agitation and careful biosafety processes (Lopes et al., 2020). Finally, the Trichoderma microcapsules were washed with distilled water with 10 ml of the sample and placed in glass Petri dishes in an oven at 30 °C for 30 minutes for the drying process (Fernández-Sandoval et al., 2012).
Table 1. Conditions for the microencapsulation of Trichoderma
Essays | Spray height | rpm | Time/min | Conc. Spores |
---|---|---|---|---|
1 | 25 | 130 | 20 | 1x108 |
2 | 35 | 120 | 30 | 1x108 |
3 | 45 | 135 | 35 | 1x108 |
The antagonistic capacity of Trichoderma spp., microcapsules
The antagonism tests began with the seeding of the pathogen at one end of the Petri dish with a disk with a 0.5 cm diameter for ten days at 26 °C in a PDA medium. After this time, strains of the beneficial fungus composed of microcapsules of T. ghanense (C4B) and T. reesei (C2A) were placed at the other end of the plate, and 0.5 cm discs with both Trichoderma strains were used as a positive control. The samples were incubated at 26 °C for three days, tests were performed in triplicate, and growth data were taken every 24 hours (Galarza et al., 2015). To estimate mycoparasitism, macroscopic observations of the dual cultures were made, considering the mycoparasitism index and the antagonist's invasion on the pathogenic mycelium's surface (Ezziyyani et al., 2004). Additionally, parameters such as the Radius of Antagonistic Growth (RCA), Radius of Pathogenic Growth (RCP), Mycoparasitism (MICMO), and Percentage of growth inhibition (PIRG) were considered.
Cocoa hand pollination
In the experiment carried out in the field, local cocoa varieties were pollinated by hand at the INIAP Boliche Experimental Station. The flowers were selected based on their opening and the distance between the trees; a random selection was made. Finally, the flower petals were carefully removed to avoid incompatibilities and to access the pollen anthers (Toledo-Hernández et al., 2020).
Biological control analysis on M. roreri under semi-controlled conditions
In the evaluation of T. ghanense against M. roreri, pathogenic strains of 11 days of growth were used. For the spore collection process, 4 ml of sterile water was added to the Petri dish, shaking gently with a handle until the suspension was diluted ten times. This dilution prepared a spore solution of the pathogen at a 1x106 spores/ml rate, verified by counting in a Neubauer chamber. Likewise, a spore suspension was prepared for the beneficial fungus and the microcapsules with a 1x108 spores/ml concentration. On the other hand, the treatment with dry Trichoderma microcapsules was diluted again in a volume of water (1:1 v/v) for field application (Krauss et al., 2010; Mbarga et al., 2014).
Table 2 Description of Trichoderma treatments for application in 15-day-old fruits
N° fruits | N° treatments | Treatments |
---|---|---|
4 | 1 | Application of microcapsules of T. ghanense and pathogen (MCP). |
4 | 2 | Dried microcapsules of T. ghanense and the pathogen (MCPs). |
4 | 3 | T. ghanense spore solution and the pathogen (S.Esp). |
4 | 4 | Positive control (Control +). |
4 | 5 | Negative control (Control -). |
In the first trial, 15-day-old fruits were used, and each treatment of T. ghanense included the positive and negative control (Table 2). The applications carried out in this test were simultaneously between the pathogen M. roreri and the aqueous microcapsules of T. ghanense (MCP). In the same way, we treated dry microcapsules of T. ghanense (MCPs) as the positive control, which was the T. ghanense spore solution (S.Esp). After making the different applications, the fruits were covered with transparent polyethylene covers, and a humidified paper towel was placed inside the cover to maintain high relative humidity (Mbarga et al., 2014).
The second experimental trial at 28-day-old fruits had six treatments, including positive and negative controls (Table 3). For field inoculation in the first treatment, the aqueous microcapsules of T. ghanense were applied, and five days later, the pathogenic strain M. roreri (MCP+MR) was used on the same fruit. The beneficial strain's spore solution (S.Esp) was inoculated, and five days later, the pathogenic strain (S.Esp + MR) was. On the other hand, following the inoculations, other pods were inoculated with the pathogenic strain of M. roreri in the first instance, and after five days, the beneficial strain both in microcapsules (MR + MCP) and in spore solution (MR + S.Esp) (Sriwati et al., 2019).
Table 3 Description of Trichoderma treatments for application in 28-day-old fruits
N° fruits | N° treatments | Treatments |
---|---|---|
3 | 1 | Application of microcapsules of T. ghanense and pathogen (MCP+MR). |
3 | 2 | Pathogen application, then T. ghanense microcapsules (MR+MCP). |
3 | 3 | Application of T. ghanense in spore solution after the pathogen (S.Esp+MR). |
3 | 4 | Pathogen application, then T. ghanense spore solution (MR+S.Esp). |
3 | 5 | Positive control M. roreri (Control +). |
3 | 6 | Negative control - water (Control -). |
Biological control analysis of microcapsules against M. roreri in commercial plantation
The analysis was carried out for three months on a commercial farm in the Taura de Naranjal parish, Guayas province. The study carried out in this plantation was with the strain T. ghanense (C4B) due to the effects described in previous experiments. Three treatments were applied, as described in (Table 4). The concentration of the beneficial strains was 1x108 spores/ml, with evaluations of healthy and diseased fruits every fifteen days and simultaneous applications of the treatment with a manual pump (Leiva et al., 2020).
Analysis of data
The assumptions of normality of the treatments under study were evaluated during semi-controlled conditions and in field applications. Graphs and non-parametric analyses were evaluated with InfoStat 2018, IBM SPSS Statistics 25, and SigmaPlot 12.0 software.
Table 4 T. ghanense treatments for application in commercial cocoa farms
N° of trees | N° treatments | Treatments |
---|---|---|
10 | 1 | T. ghanense washed microcapsules (MCP). |
10 | 2 | T. ghanense spore solution (S.Esp). |
10 | 3 | Control (water). |
3. Results and discussion
Mass-microencapsulation system from T. ghanense and T. reesei
This study successfully developed the biofor-mulation with 1.5% sodium alginate and 2% calcium acetate with the beneficial strains. We improved a successful process, and as a result, the micro-encapsulation tests showed that the ideal conditions in the process were at a spray height of 35 cm, 120 rpm, for 30 minutes, and a concentration of 1x108 spores/ml in laboratory conditions. The drying time of the microencapsulated was 30 minutes at 40 °C; the formed microcapsules were visualized and characterized under a microscope (Figure 1).
Trichoderma spp., microcapsules antagonistic capacity
Percentage inhibition of mycelial growth against M. roreri cultivated with T. ghanense in a sporulated disc, with two types of microcapsules preserved in aqueous solution (MCP) and dry microcapsules (MCPs), for T. ghanense in MCP, MCPs and disc it was 85.5%, 75.9% and 74.4% respectively. In contrast, for T. reesei in MCP disc and MCPs, it was 83.9%, 76.4%, and 73.8%, respectively (Figure 2).
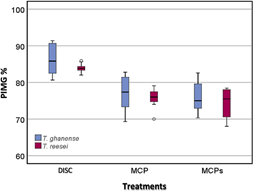
Figure 2 Box diagram of the Percentage Inhibition of Mycelial Growth (PICR) of M. roreri against T. ghanense and T. reesei, under in vitro conditions, during ten evaluation days.
The antagonistic effect of the strains of T. ghanense and T. reesei showed a high percentage of inhibition of the pathogen, considering that the differences are not significant (p = 0.427) between the strains, but between the treatment of the Trichoderma strains using sporulated disc (p = 0.0001). In addition, the dried microcapsules and the aqueous solution of T. ghanense and T. reesei did not undergo significant modifications in the biological control mechanism despite being subjected to the stress of the microencapsulation process.
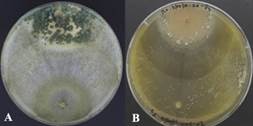
Figure 3 (A) Mycoparasitism of T. ghanense and (B) T. reesei, according to their antagonistic capacity, against the pathogen M. roreri under in vitro conditions.
It was observed that T. ghanense was superior in mycoparasitism over M. roreri since, during the ten days of evaluation, a high level of sporulation could be evidenced invading the surface of the pathogen colony under microcapsules in aqueous solution, dry microcapsules, and the disk condition (Figure 3A).
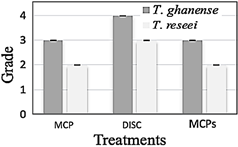
Figure 4 During ten days of evaluation, the Mycoparasitism activity of T. ghanense and T. reesei against the pathogen M. roreri under in vitro conditions.
On the other hand, microcapsules in aqueous solution and dried microcapsules of T. reesei colonized only half of the pathogen's surface (Figure 3B). Furthermore, the sporulated disc strain of T. reesei completely invaded the pathogenic colony but did not sporulate on it. In vitro tests, it was determined that the T. ghanense strain has a greater capacity to successfully use the mechanism of action of mycoparasitism than the T. reesei strain (Figure 4).
Biological control analysis on M. roreri under semi-controlled conditions
Biocontrol action by dry microcapsules (MCPs) and spore solution (S.Esp) of T. ghanense did not show high efficacy against M. roreri, unlike the aqueous microcapsules solution of T. ghanense (MCP), which did not show external damage to the fruits during the evaluation. Symptoms ranged from 1% to 25% in the favorable control fruits (M. roreri) after six weeks of assessment (Figure 5A). Additionally, the external severity evaluated in the week eighth, MCP showed 0% affectation according to grade zero of the scale (healthy fruits), S.Esp and MCPs were affected between 1% - 25% according to grade 1 of the scale, external severity without significant diffe-rence compared to the negative control (Figure 5B).
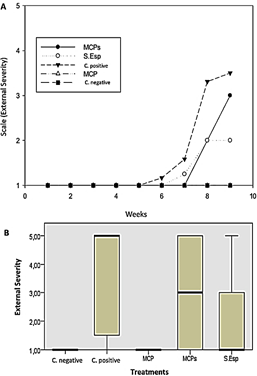
Figure 5 (A) External average severity with T. ghanense treatments during nine weeks. (B) Box plot of external severity for treatments. According to Kruskal-Wallis (p ≤ 0.94), there is no significant difference.
In this sense, it is notable that the outward symptoms, meaning the presence of a lump on the surface of the fruits, are more evident from the seventh to the ninth week (Figure 6).
The internal severity of the fruits was evaluated, ratifying the biological control action of the MCP treatment of T. ghanense on the pathogen, obtaining 0% of affected fruits, having a significant difference concerning the positive control (70%) (p = 0.002), according to damage scale 0-5. The treatments of T. ghanense S.Esp (25%) and MCPs (50%) did not show significant differences according to the damage scale (1 - 3) (Figure 7- Figure 8).
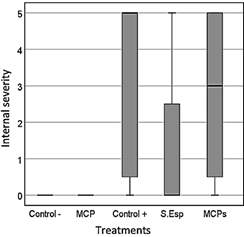
Figure 7 Average internal severity in the ninth week after inoculation of the pathogen and antagonist.
Regarding external severity, it was evidenced that the MR+S.Esp, MCP+MR, and MR+MCP treatments were significantly affected (p = 0.03) compared to the negative control, with values between 26% and 75% according to grades three and four on the severity scale. However, the S.Esp+MR treatment was not significantly affected (p = 0.620) compared to the negative control, with values between 1 and 25% according to grade 2 of the scale (Figure 9).
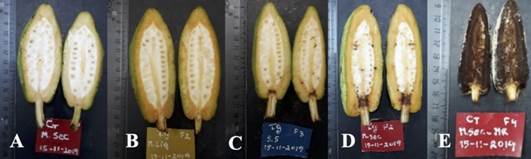
Figure 8 Internal aspect of cocoa fruits inoculated with M. roreri and different formulations of T. ghanense in fruits at 15 days of age. (A) negative control (water), (B) microcapsule with an aqueous solution of T. ghanense and pathogen (MCP), (C) solution of spores of T. ghanense and pathogen (S.Esp), (D) dried microcapsules of T. ghanense and pathogen (MCPs) and (E) positive control (pathogen).
The internal severity, MR+S.Esp, MCP+MR, and MR+MCP treatments showed a significant affectation between 81% to 100% according to the damage scale 4-5, in contrast to the negative control, while S.Esp+MR was not significantly affected, presenting damage between 41% and 60% according to degree three of damage compared to the negative control (Figure 10).
Biological control of T. ghanense microcapsules in a commercial plantation
In evaluating a commercial cocoa plantation, neither the microcapsules nor the spore solution significantly reduced the disease already present in the plantation to the control, as observed in the analysis of the area under the curve (Figure 11).
Analysis of the biological control of T. ghanense microcapsules in a commercial plantation
During the evaluation of the biological control activity of the microcapsules under field conditions, the high values correspond to the most affected treatments according to the area under the disease progress curve (ABCPE). In this sense, the applications of the liquid solution of T. ghanense microcapsules (MCP) and spore solution (S.Esp) did not significantly decrease the incidence of the disease compared to the control treatment (water) (Figure 12).
On the other hand, with the applications made at the evaluation time, it was observed that the number of healthy fruits after the application increased in the treatment with the microcapsule solution and spore solution to the control obtaining. As a result, an increase of 98.84%, 90.925%. and 81.73%, respec tively (Figure 13).
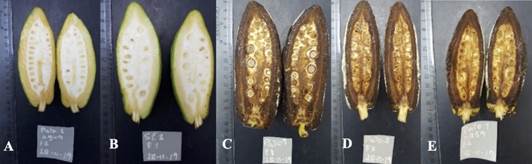
Figure 11 Internal aspect of cocoa fruits inoculated with M. roreri in 28-day-old fruits. (A) Negative control (water), (B) Treatment of the interaction of T. ghanense spore solution and pathogen (S.Esp+MR), (C) Microcapsules in aqueous solution of T. ghanense and pathogen (MCP+MR), (D) Pathogen and dried T. ghanense microcapsules (MR+MCP), and (E) Positive control (MR).
The encapsulation or microencapsulation process has been studied and applied with different microorganisms, like Lactobacillus plantarum, improved its viability using an external ionic gelling method, using three coating materials: 2% alginate (Al), 1% alginate plus 1% gellan gum (Al-GG) and 1.5% alginate plus 3% gum Arabic (Al-GA) by the atomizer, thus showing improvements in the storage stability of the cells (Chun et al., 2014).
Trichoderma asperellum extract was developed microcapsules using 20 ml, which consisted of a mixture with 80 ml of 2% sodium alginate previously sterilized, left stirring for 15 min, and sprinkled on a container that contained 2% Calcium Chloride (CaCl2), forming the microcapsules instantly (Lopes et al., 2020).
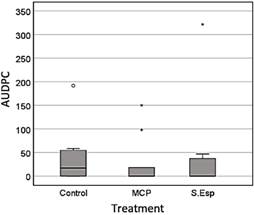
Figure 12 Average disease incidence through the area Under the Disease Progress Curve (AUDPC) during the 90 days of evaluation, with no significant difference between treatments, according to the Kruskal-Wallis test (p ≤ 0.05).
The Trichoderma genus has been qualified as a biological control agent for diseases such as M. roreri and M. perniciosa, both under in vitro and in vivo conditions in several geographical locations (Mejía et al., 2008; Leiva et al., 2022). Trichoderma spp., presents great antagonistic potential on mycelial growth when competing for space with the phytopathogenic fungus (Suárez Meza et al., 2008).
Additionally, T. asperellum co-encapsulated with chitosan showed a PIC of 40% against M. roreri, superior to the non-encapsulated control, evidencing favorable advantages for field applications (Carrera et al., 2017). Likewise, the T. harzianum strain overgrew, surpassing the growth of the pathogen Fusarium oxysporum, showing a percentage of inhibition more significant than 50% (Fernández & Suárez, 2009).
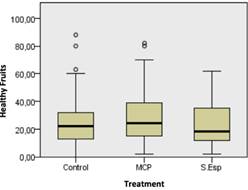
Figure 13 The number of healthy fruits during 90 days of evaluation shows no significant difference according to the Kruskal Wallis test (0.05).
According to (Mejía & Alvarado, 2016), Trichoderma spp., showed a range between 40.13% to 46.77% of PICR against four M. roreri isolates was evidenced that T. ghanense (C4B) and T. reesei (C2A) in microcapsules obtained 77.4% and 76.4% growth inhibition against M. roreri, which suggests the ability to control the pathogen at a level in vitro, turning them into candidate strains for the biological control of diseases.
On the other hand, the effects of Trichoderma mycoparasitism against M. roreri have shown a deformation of the hyphae of the pathogen; due to this action of the beneficial strain, the pathogen was not able to grow when replanted again (Suárez Meza et al., 2008). In this context, it was determined that the microcapsules of the T. ghanense strain produce a total invasion of the colony surface of the phytopathogenic fungus M. roreri. Only T. ghanense grew and sporulated on the pathogen; however, the strains showed no significant differences. In the same way, in previous investigations, they mention that the strains of Trichoderma spp., colonize, coil, and parasitize hyphae of Moniliophthora spp. and Phytophthora spp. as mechanisms for biocontrol (Mejía et al., 2008; Loguercio et al., 2009). Trichoderma strains have shown that they exert antagonism through the mechanisms of antibiosis and mycoparasitism ac tion against P. infestans, Sclerotium rolfsii, Phytophthora spp., Rosellinia spp, F. oxysporum, causing pathogen mycelium lysis (Jaimes & Aranzazu, 2010).
On the other hand, it has been shown that non-encapsulated microorganisms (e.g., Lactobacillus delbrueckii) lose their viability after 180 minutes of environmental exposure (Cuello et al., 2015).
However, the in vitro tests carried out in this work demonstrated the efficacy of the T. ghanense and T. reesei strains despite having gone through the stress generated by the microencapsulation process. In this sense, viability likely decreased by a certain percentage, but the strains grew and generated biocontrol against the pathogen, even managing to expand on it.
4. Conclusions
For the microencapsulation of T. ghanense and T. reesei, with sodium alginate and calcium acetate, it was determined that the ideal conditions were at 120 rpm, for 30 minutes, at the height of 35 cm and a spore concentration of 1x108, for an adequate uniformity of the microcapsules.
The washed microcapsules and the dried microcapsules of T. ghanense (C4B) and T. reesei (C2A) did not undergo significant modifications despite having been subjected to physical and chemical stress in the process, so it is convenient to use them as a control biologically, in the inhibition of the pathogenic fungus M. roreri (MR59).
The washed and dried microcapsules of T. ghanense and T. reesei present an adequate level of mycoparasitism, which indicates that these strains are an alternative for the biological control of the pathogenic fungus (M. roreri) carrying constant cycles of applications. Under field conditions, the microcapsules of T. ghanense show a higher percentage of antagonism against M. roreri, even when the pathogen was applied together with the antagonist, in fruits of approximately 15 days.
In applying the pathogen-antagonist and anta-gonist-pathogen combinations in 28-day-old fruits, almost all the treatments were affected (81%-100%), except the treatment with spore solution (S.Esp), where the damages were minor at 60%. According to the data obtained, it is suggested that the application of microcapsules of T. ghanense be carried out fifteen days after the formation of the fruit.
With three applications of T. ghanense micro-capsules every fortnight, by spraying cocoa plantations with a pump, a greater quantity of healthy fruits could be obtained in commercial farms. The application of microcapsules based on Trichoderma strains at early ages generates protection against Moniliophthora roreri throug-hout the vegetative development of cocoa.
As part of future research work, the discovery of new biological substances that protect the spores during the application process and adaptability to environmental conditions and that can cope with the various phytopathogenic fungi that destroy a wide variety of crops will be performed, which will allow an increase in commercialization, yield and use, both industrially as well by small producers of Trichoderma for biocontrol.