Services on Demand
Journal
Article
Indicators
-
Cited by SciELO
Related links
-
Similars in SciELO
Share
Revista de la Facultad de Medicina Humana
Print version ISSN 1814-5469On-line version ISSN 2308-0531
Rev. Fac. Med. Hum. vol.20 no.4 Lima Oct-Dec 2020
http://dx.doi.org/10.25176/rfmh.v20i4.3004
Review article
Electrospinning: Advances and applications in the field of biomedicine
1Laboratorio de Biotecnología Animal
2Facultad de Ciencias Biológicas, Universidad Ricardo Palma, Lima-Perú.
El Electrospinning (electrostatic fiber spinning) is a modern and efficient method that uses the electric field to produce fine fibers that manufacture porous and versatile structures called scaffolds organized by nanofiber units. A descriptive study was carried out, reviewing databases such as MeSH, NCBI, Nature, NIH, PloSONE, ReseachGate, from which we selected a total of 28 articles. This work explains a review of the electrospinning process, its products, and applications such as the inoculation of treatment or prophylaxis drugs, tissue regeneration, determining the importance of collective knowledge of all associated factors in tissue bioengineering. The development of electrospinning technology research has proven to be of great interest in biomedicine. To promote biomedical engineering in Peru, this type of progress must be supported and encouraged, which seeks to benefit the population’s health.
Key words: Electrospinning; Scaffolds; Nanofiber; Tissue Engineering (Source: MeSH NLM).
INTRODUCTION
The field of biotechnology has different methodologies applied to cell regeneration, proliferation, and culture. It seeks to use new technologies and complement them with characteristics that allow their application in medicine and human health.
The electrospinning technique combines biology and printing technology to create nanofiber in “scaffolds” that provide support similar to the extracellular matrix’s fibrous protein. For the creation of nanofiber, organic solvents and acids are used which, due to their molecular interaction, allow the formation of a defined nanofiber structure; However, the use of these products denatures proteins, growth factors1and can alter and inhibit the production of other intrinsic components of cells for their development.
Natural polymers provide properties that mimic biological functions such as cell signaling, but have no control over their structural characteristics, such as fiber diameter. On the other hand, synthetic polymers allow control of the structure. However, the signaling capacity decreases2. Collagen from animals has been widely studied as a biomaterial for the production of the nanofiber. However, other materials such as alginate or aloe vera have not been studied in-depth, although they may be more useful since they are less toxic to cells inserted in scaffolds than synthetic materials. The product must have the necessary characteristics for cell proliferation and structuring to properly couple to nanofibers and culminate in tissue formation suitable for implantation1.
PVA is a semi-crystalline, highly hydrophilic, non-toxic, and biocompatible polymer, with properties of resistance, solubility in water, permeability to gases, and thermal characteristics. The degree of hydrolysis directly affects the nanofiber’s resistance. Since PVA is a very hygroscopic element, as it contains a more significant amount of water or humidity, it reduces its mechanical capacity3.
Factors such as voltage, surface tension, electrical conductivity, a molecular weight of the polymer, and volatility of the solvent intervene in the morphology and structuring of nanofibers.
In this review, we will analyze the use of Electrospinning technology and the parameters that influence nanofiber production, the different biomaterials, and the applications in nanopharmaceuticals and the production of scaffolds.
ELECTROSPINNING TECHNIQUE
It allows the production of polymeric fibers with diameters varying between at least 3 nm and 5 μm4. It consists of an electrical mechanism that can be managed by different variables; according to the Doshi and Reneker classification, these are divided into solution, properties, controlled variables, and environmental parameters.
The electrospinning technique. The assembly of the assembly consists of three main components: a high voltage source that has two electrodes which are connected to the output of the metal needle and another directly to the collector plate, a metal needle, and a collector plate (conductive metal sheet). An infusion pump is used to drive the polymer solution through the capillary to the collecting plate. The electric field force overcomes the drop’s surface tension that forms at the end of the needle, and the drop is distorted, forming the Taylor cone. This distortion causes the expulsion of the electrically charged polymer towards the collector, creating thin threads. If the collector is rotating, it is possible to prepare aligned polymeric fibers5.
The control of the different parameters provides unique characteristics to the fiber obtained by electrospinning, so the execution process is critical. It should be noted that for each polymer and solvent used, their dissolution parameters will be different.
Solution parameter
The concentration of the polymeric solution: The size and morphology of the fiber are directly related to the concentration of polymers, influencing the viscosity associated with the entanglement of the polymeric chains, the lower the entanglement, the lower the density. If the concentration is very dilute, the fibers break before reaching the collector plate due to the surface tension. On the contrary, if it is highly concentrated, the fiber will not form due to its high viscosity, impeding the passage of the solution through the capillary.
The dissolution conductivity: When salts are added to the solution, the conductivity increases, and therefore the electrical force for the stretching of the fiber, directly influences the diameter of the fiber.
Dielectric effect of the solvent: The solvent fulfills two essential functions in the electrospinning process to dissolve the polymer molecules to form the jet with an electrical charge, and the second is to carry these same dissolved polymer molecules to the collecting tube.
Processing parameters
Voltage: Main parameter, since only a voltage that exceeds the threshold, can generate the Taylor cone that will be expelled towards the collector tube. This high voltage will provide more significant stretch, promoting a reduction in the diameter of the nanofiber.
Outflow: When the flow is larger in diameter, it increases the size of the fiber, producing defects in it. On the contrary, a minimum value of the outflow diameter would help keep the Taylor cone stable by allowing time for the evaporation of the solvent before reaching the collecting tube.
Distance between the tip of the needle and the collector plate: The variation in the distance between the needle and collector may or may not affect the thickness and shape of the fiber, causing the appearance of beads, lumps, or wet fibers. A small distance will prevent the solvent from evaporating and the polymer coming out as a string. When working with a much greater distance, the fiber could break due to its weight.
An optimization method with the most relevant parameters, such as the needle-collector distance, output flow, voltage, and concentration of the solution, is essential6.
Biomaterials
Polymeric fibers produced from polymer solutions have intrinsic optical guidance properties, as well as specific mechanical conditions.
Biomaterials are classified into biodegradable and non-biodegradable materials. They can be used depending on the release, if it occurs only by diffusion or by diffusion and degradation of the scaffold simultaneously.
Nanofibers come primarily from biodegradable polymers such as polylactic acid (PLA), polycaprolactone (PCL), poly (lactic-co-glycolic acid) (PLGA), and polyethylene oxide (PEO). In the same way, Electrospinning has been carried out with natural polymers such as chitosan, collagen, hyaluronic acid, cellulose, silk, and other materials, among which it is possible to name PLLA, PEG(7).
Natural Polymers:
Collagen Scaffolds
Collagen is a trimeric molecule that consists of three polypeptide α chains; these intertwine in a triple helix to form homotrimers or heterotrimers. Triple helical sequences are composed of Gly-XY repeats, X being frequently proline, and Y often 4-hydroxyproline. Each collagen contains at least one triple-helical domain (COL) located in the ECM, as well as non-collagenous (non-Gly-XY) regions (NC domains).
The intercalation of COL domains between NC domains makes collagen a multi-domain protein. NC domains participate in the structural assembly and provide collagen with biological activities. To date, 28 different types of collagen have been identified. Collagen types I, II, and III are the most common collagens and are called classic fibril-forming collagens8. The intercalation of COL domains between NC domains makes collagen a multi-domain protein. NC domains participate in the structural assembly and provide collagen with biological activities. To date, 28 different types of collagen have been identified. Collagen types I, II, and III are the most common collagens and are called classic fibril-forming collagens9.
Hyaluronic Acid Hyaluronan
An abundant polysaccharide is found almost exclusively in the joints, allowing cells to bond together for cartilage regeneration. Hyaluronic Acid is a polymer associated with aqueous solutions characterized by high levels of viscosity. Due to this factor, the typical Electrospinning processes cannot be developed to obtain a constant jet stream successfully. For this reason, the Electrospinning methodology “blowing-assisted” is established. In this process, the sample must be rotated with the help of the airflow at high temperatures. In this way, the processing window extended8.
Cartilage repair can be applied for Hyaluronic Acid electrospun nanofiber scaffolds with a convenient degree of post-crosslinking. The critical parameters for processing non-woven nanofibrous membranes by electrospinning “blowing-assisted” with hyaluronic acid, using rheology and scanning electron microscopy methods. It showed that the air blowing temperature is the most useful parameter to ensure Hyaluronic acid nanofibers’ formation. As the blown air temperature increased from 25 to 57 ° C, nanofiber’s formation became consistent and uniform10.
Chitosan
Chitosan is a polysaccharide composed of acetamido 2 deoxy-β-D glucan, (N-acetyl D glucosamine and 2 amino 2 deoxy β-D glucans, which is obtained by partial deacetylation of chitin.
Chitosan is a cationic biopolymer and in Aqueous solution has polyelectrolytic effects, highly toxic organic solvents such as hexafluoroisopropanol (HFIP) or trifluoroacetic acid (TFA) denatures its properties and natural structure and further breaks the interactions between chains. However, for the production of electrospun micro or nanofibers Of chitosan, the common solvents used are trifluoroacetic acid (TFA) or solutions composed of dilute acetic acid. Another option is neutralization using alkaline compounds or cross-linkers such as glutaraldehyde and genipin, although it can cause partial or total loss of natural characteristics. It is also poorly soluble with commonly available organic solvents, which impairs the formation of continuous fibers and sets the ideal viscosity. The nature of chitosan complicates the capacity for fiber formation, affecting its properties. In this situation, it is recommended to use a low concentration of dilute acetic acid and a fiber-forming agent11.
Alginate
Alginate is a complete family of linear copolymers containing blocks of attached β-mannuronate and α-L-guluronate residues. The blocks are composed of consecutive G residues, consecutive M residues, and alternating M and G residues. Alginates extracted from different sources differ in the content of M and G and the length of each block.
It is typically obtained from brown algae (Phaeophyceae) such as Laminaria Hyperborea or by bacterial biosynthesis. Bacterial alginate can be produced from Azotobacter and Pseudomonas. It has been widely investigated and used for many biomedical applications, due to its biocompatibility, low toxicity, relatively low cost, and gentle gelling. Furthermore, alginate gels also show promise for cell transplantation in tissue engineering12.
Phospholipids
Lecithin is a natural mixture of phospholipids and neutral lipids. It forms cylindrical inverse micelles in non-aqueous solutions. As the concentration of lecithin rises in solution, the micellar morphology changes from spherical to cylindrical. At higher concentrations, cylindrical micelles overlap and entangle like that of polymer chains in semi-dilute or concentrated solutions. Water and other polar molecules serve to bind the phosphate head groups between phospholipids.
Phospholipid electrospun fibers such as lecithin, with diameters of 1 to 5 microns, offer the potential for direct fabrication of high-surface, biological membranes without multiple synthetic steps, or post-processing electrospinning surface treatments13.
Synthetic Polymers:
Electrospun synthetic polymers include Polyglycolic Acid (PGA), Polyglycolic Lactide (PLGA), Polycaprolactone (PCL), Polyurethane (PU), Polylactic Acid (PLA), Polystyrene (PS), and Polyvinyl Alcohol (PVA).
Synthetic copolymers
The use of copolymers is a viable scheme to generate new materials with desirable properties. When properly implemented, the performance of electrospinning scaffolds based on copolymers can be significantly improved compared to homopolymers. For example, biodegradable hydrophobic polyesters generally have good mechanical properties, but lack cellular affinity for tissue engineering. The incorporation of a suitable hydrophilic polymer segment can increase cellular affinity. In addition to cell affinity, mechanical properties, morphology, structure, size, and distribution of pores, biodegradability, and other physical properties can also be tailored through the use of copolymers. With amphiphilic copolymers as protective molecules to encapsulate drug molecules, electrospun scaffolds can be used for drug delivery in a controlled manner8.
Polymer Blends
The blends or blends have an advantage over copolymers in that synthetic schemes do not limit them. Therefore, nanofibrous scaffolds formed by mixing different polymers between natural and synthetic, become an attractive option, especially for natural polymers, which present more complications when modifying their internal structure to obtain fibers. These polymers are the most suitable for biomedical applications.
Polymer Blends
Collagen-Chitosan (CC)
The Collagen-Chitosan (CC) blend can modify mechanical and biological properties to mimic the natural tissue extracellular matrix (ECM). The addition of chitosan modifies collagen’s helical characteristics, changing the physical properties by introducing additional hydrogen bonds.
The various factors affecting the tensile behavior of electrospun CC biomaterials, such as individual fibers and fibrous membranes, have been investigated in detail. In terms of the CC blends’ fiber morphology, the fiber diameter decreases with increasing chitosan content. These fibers would present higher tensile strength in contrast to the larger diameter fibers.
In the case of electrospun DC membranes, the increase in final tensile strength is observed as the chitosan content decreases. The mechanical properties depend on the fiber’s diameter and collagen ratio to chitosan in the fibers12.
Blend of Natural and Synthetic Polymers
Natural Polymers generally have weak mechanical properties; however, the blend between natural and synthetic polymers combines the favorable characteristics such as the synthetic polymer’s strength and durability and the specific cellular affinity of a natural polymer. Therefore, electrospun scaffolds based on natural and synthetic polymers’ blends improve both the physical properties and the fibers’ biological functionality.
PLGA with PEG-g-CHN (Chitosan)
The addition of PEG-g-Chitosan lowers the glass transition temperature of PLGA, resulting in a decrease in tensile strength at break and an increase in scaffold pressure.
Composite PLGA / chitin scaffolds perform better than pure PLGA scaffolds in human keratinocytes. The unique structure of PEG-g-Chitosan has also shown the ability to release controlled hydrophilic drugs from electrospun PLGA scaffolds. Studies have been carried out using mixtures of PEG-g-CHN and PLGA with different proportions to manufacture electrospinning scaffolds for drug release, observing the significant decrease in the drug’s initial release rate from the scaffold and the prolonged-release time. According to the released release rate, it is concluded that PLGA polymer scaffolds with PEG-g-CHN, mechanically strong and compatible, could be suitable candidates for biomedicine applications, specifically in surgical prevention treatments8.
PLGA with PEG-PLA copolymers
The combined advantages of composition adjustment, drug loading capacity and manageability, and biocompatibility are delivery; since the material is relatively hydrophobic, they rank these scaffolds as potential candidates for further clinical applications and evaluations.
To prevent surgery-induced adhesion, the potential use of a scaffold composed of PLGA, PEG-PLA diblock copolymer, and cefoxitin sodium was evaluated. This medicated PLGA electrospun-based scaffold was able to completely prevent any adhesion formation after 28 days, with physical barrier properties, but with the ability to deliver drugs14.
Applications in biomedicine
Scaffolds design
The increase in new biomaterials obtained by the Electrospinning technique has different types of applications derived from their unique properties, such as their high surface/volume ratio, porosity, physicochemical properties, and the versatility of the technique. In the development of microfiber(15). In the last decades, the most frequent application for this technique is cell support or substrate "scaffolds" in tissue engineering. The scaffolds allow to control and adapt the structure’s anatomy in which the support’s external shape must be adequate to the tissue’s needs. The nanofiber can be inoculated with nutrients, growth factors, or genes that stimulate tissue regeneration. Scaffold applications have been made in the biomedical area to form cartilage, bones, arteries, and skin and nerve regeneration(Figure N.º 2)
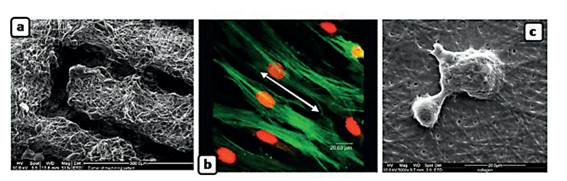
Figure N.° 2 a) Preparation of support from electrospun nanofibers of polycaprolactone (PCL). b) Support from aligned polyacid lactic (PCL) fibers for the growth of coronary cells c) Support from electrospun collagen fibers in a stem cell culture.15
The accumulation of free radicals in our cells is related to neurological disorders, cardiovascular diseases, and cancer. Therefore studies have been implemented to capture free radical molecules using nanofibers with antioxidant agents16. In this same study, cancer cells were treated by developing nano convectors that, using imaging agents, allow them to be directed at tumors.
Bone Tissue Engineering
Diseases related to bone tissue cause structural damage to the surface of the bone’s articular cartilage, causing severe pain and disability to millions of people worldwide. It is known that medical treatment is aimed at alleviating the damage, but not at improving the injuries. It is because of this that a specialized technique is needed in bone reconstruction. The calcium phosphate (CaP) nanostructure is a biomaterial used to manufacture scaffolds in bone regeneration, since it mimics the organic component of bone. One of the applications of (CaP) is its use as a nanostructure. It combines natural or synthetic polymers with CaP nanostructures. This compound is used as a successful strategy, since bone itself is a nano component of HA and Collagen, whose composition contains (CaP). Another exciting application of CaP is its interaction with stem cells. It is an ideal nanomaterial for orthopedic repair, since its level of action goes beyond a simple filling for bone. It serves as scaffolds providing support for cell adhesion in which it gives chemical and mechanical signals and regulates cell behavior promoting the bone regeneration process.
CONCLUSION
Natural and synthetic polymers are used in combination to manipulate and take advantage of material properties such as thermal stability, mechanical strength, and barrier properties, depending on the specific application.
The diameter of the fiber obtained by electrospinning depends on the solution parameters, the most important being: the concentration, viscosity, conductivity of the polymer solution, and the dielectric effects of the solvent, to this we add the process variables, electric field intensity, voltage, output flow and working distance between the needle tip and the collecting tube; These parameters determine the obtaining of specific and desired nanofiber, or on the contrary, with beats, lumps or wet fibers, which are neither required nor viable in the design of nanostructures.
The use of nanofibers is an innovative technology that allows the creation of Scaffolds structures with unique properties and characteristics highlighting their porosity, which makes it essential to produce new applications in the area of biomedicine, textiles, and food. The creation of these technological advances allows increasing production using a variety of biomaterials while reducing costs.
REFERENCES
1. Aoki, H, Miyoshi H, Yamagata Y. Electrospinning of gelatin nanofiber scaffolds with mild neutral cosolvents for use in tissue engineering. Polymer Journal. 2014; 47(3), 267-277. https://doi.org/10.1038/pj.2014.94. [ Links ]
2. Jenkins T, Little D. Synthetic scaffolds for musculoskeletal tissue engineering: cellular responses to fiber parameters. Npj Regenerative Medicine. 2019; 4(15),1-14. https://doi.org/10.1038/s41536-019-0076-5 [ Links ]
3. Park J, Takeru K, Kwan K, Byoun K, Myung K, et al. Electrospun poly(vinyl alcohol) nanofibers: Effects of degree of hydrolysis and enhanced water stability. Polymer Journal. 2010; 42(3), 273-276. [ Links ]
4. Pham P, Sharma U & Mikos G. Electrospinning of polymeric nanofibers for tissue engineering applications: A review. Tissue Engineering.2006;12(5), 1197-211. [ Links ]
5. Duque L, Rodriguez L, Lopez M. ELECTROSPINNING: LA ERA DE LAS NANOFIBRAS. Revista Iberoamericana de Polímeros. 2013;14(1),10-27. [ Links ]
6. Li D & Xia Y. Electrospinning of Nanofibers: Reinventing the Wheel? Advanced Materials.2004; 16(14), 1151-1170. [ Links ]
7. Pataquiva A & Coba S. Producción de nanofibras poliméricas mediante el proceso de electrospinning y su uso potencial. Mutis.2018; 8(1), 17-33. [ Links ]
8. Liang D, Hsiao S. & Chu B. Functional electrospun nanofibrous scaffolds for biomedical applications. Advanced drug delivery reviews. 2007;59(14), 1392-1412 [ Links ]
9. Dong C & Lv Y. Application of collagen scaffold in tissue engineering: recent advances and new perspectives. Polymers.2016; 8(2), 42. [ Links ]
10. Wang P, Zhao L, Liu J, Weir M, Zhou X, Xu H. Bone tissue engineering via nanostructured calcium phosphate biomaterials and stem cells. Bone Research. 2014; 2, 14017. [ Links ]
11. Qasim S, Zafar M, Najeeb S, Khurshid Z, Shah A, Husain S, et al.Electrospinning of chitosan-based solutions for tissue engineering and regenerative medicine. International journal of molecular sciences.2018; 19(2),407 [ Links ]
12. Lee K & Mooney D. Alginate: properties and biomedical applications. Progress in polymer science. 2012;37(1),106-126. [ Links ]
13. McKee M, Layman J, Cashion P. Phospholipid nonwoven electrospun membranes. Science. 2006; 311(5759), 353-355. [ Links ]
14. Zong X, Li S, Chen E, Garlick B, Kim K-S, Fang D, et al. Prevention of postsurgery-induced abdominal adhesions by electrospun bioabsorbable nanofibrous poly(lactide-co-glycolide)-based membranes. Annals of surgery. 2004; 240 (5), 910-915. [ Links ]
15. García, N. Electrospinning: una Técnica Fascinante para la Obtención de Nanofibras Poliméricas. Revista de Plásticos Modernos. 2013; 105, 166. [ Links ]
16. Garcia-Robles M, Rodríguez-Félix F, Márquez-Ríos E, Barrera-Rodríguez A, Aguilar-Martinez J, Del-Toro-Sánchez C. Aplicaciones Biomédicas, Textiles y Alimentarias de nanoestructuras elaboradas por electro hilados. Revista de Ciencias Biológicas y de la Salud. 2014; 16(2), 44-52. [ Links ]
17. Ghosal K, Chandra A, Praveen G, Snigdha S, Roy S, Agatemor C, Sabu T, Provaznik I.. Electrohilado sobre fundición con disolvente: ajuste de las propiedades mecánicas de las membranas. Sci Rep. 2018; 8, 50-58 https://doi.org/10.1038/s41598-018-23378-3 [ Links ]
18. Calzón Gutiérrez A. Desarrollo de un equipo de electrospinning para obtención de nanofibras alineadas de recombinámeros tipo elastina (Grado en Ingeniería Mecánica). Universidad de Valladolid; 2016. [ Links ]
19. Lim T. Nanofiber technology: current status and emerging developments. Progress in Polymer Science. 2017; 70, 1-17. [ Links ]
20. Matysiak W, Tanski T, Smok W. Electrospinning as a versatile method of composite thin films fabrication for selected applications. Solid State Phenomena. 2019; 293, 35-49. [ Links ]
21. Mengistu Lemma, S., Bossard, F., & Rinaudo, M. Preparation of pure and stable chitosan nanofibers by electrospinning in the presence of poly (ethylene oxide). International journal of molecular sciences. 2016; 17(11), 1790. [ Links ]
22. Moon S, Gil M, Lee K. Syringeless electrospinning toward versatile fabrication of nanofiber web. Scientific Reports (Nature Publisher Group). 2017; 7, 41424. [ Links ]
23. Olvera-Gracia. M, Aguilar-Hernández J, Kryshtab Tetyan. Procesamiento de micro y nanofibras de polipirrol/óxido de polietileno/nylon-6 por la técnica de electrohilado. Ingeniería Investigación y Tecnología. 2013; 14(4), 575-585. [ Links ]
24. Park Y, You M, Shin, J, Ha S, Kim D, Haeng, Heo M, Nah J, Kim Y, Seol J. Thermal conductivity enhancement in electrospun poly(vinyl alcohol) and poly (vinyl alcohol)/cellulose nanocrystal composite nanofibers. Sci Rep. 2019; 9, 3026. https://doi.org/10.1038/s41598-019-39825-8 [ Links ]
25. Pérez-González GL, Villarreal-Gómez LJ, Serrano-Medina A, Torres-Martínez EJ, Cornejo-Bravo JM. Mucoadhesive electrospun nanofibers for drug delivery systems: applications of polymers and the parameters' roles. Int J Nanomedicine. 2019;14,5271-5285. [ Links ]
26. Prabhakaran MP, Kai D, Ghasemi-Mobarakeh L, Ramakrishna S. Electrospun biocomposite nanofibrous patch for cardiac tissue engineering. Biomed Mater. 2011; 6(5):055001. [ Links ]
27. Rodríguez Pérez E. Diseño de nuevos biomateriales basados en redes poliméricas interpenetradas de ácido hialurónico y polímeros acrílicos (Tesis doctoral). Universitat Politècnica de València; 2017. [ Links ]
28. Vazquez-Gonzalez J L, Cordova Estrada A K, Cordova F. Diseño Mecatrónico de un Sistema de Electrospinning para la fabricación de nanofibras a bajo costo. ResearchGate. 2017; 1,575-581. [ Links ]
29. Wendorff, J, Agarwal, S, Greiner, A. Electrospinning: materials, processing, and applications. John Wiley & Sons. 2012. [ Links ]
Received: August 16, 2020; Accepted: August 30, 2020