INTRODUCTION
Currently, the assessment of the dynamic response of complex structures such as cable-stayed bridges rely upon developing a finite element model that could represent the real structural behavior of the structure under dynamic loads. However, in addition to the variability of the dynamic excitations, the uncertainty in the material properties and the assumptions made during modeling compromise the reliability of the results obtained from the numerical models. To overcome this issue, an alternative is to compare and validate the numerical results with field measurements of the structure vibration. Over the years, field measurements are been performed along with modal identification methods (MIM) to calibrate or update the numerical models in order to accurately detect the dynamic modal parameters [1]. All this process is known as Experimental Modal Analysis (EMA) [2] [3] [4] [5] [6].
EMA uses input-output (forced vibration) or output-only (ambient vibration) techniques to generate the field-recorded data [2] [3] [4] [5]. However, several works [7-11] have demonstrated the success of ambient vibrations in determining the modal parameters of cable-stayed bridges. After recording the data, MIMs are needed to assess the dynamic parameters. Generally, these methods can be classified according to its domain of application. In case of MIMs such as: the peak-peaking method (PP) [12-17], the frequency domain decomposition method (FDD) [18] [19] [20], and the stochastic subspace identification method (SSI) [21] [22] [23] [24], they are classified as frequency-domain identification methods. In contrast, if the data is analyzed in the time-domain, MIMs such as: the random decrement technique (RDT) [25] [27] [28] and Ibrahim time domain method (ITD) [29] [30] [31] [32] [33] [34] for instance are classified as time-domain identification methods. These methods can be labelled as basis MIMs.
Since the 2000s, the popularity of cable-stayed bridges in Peru has increased in the development of bridge infrastructure projects due to its remarkable features to cover long spans in challenging geographical situations. However, an inaccurate assessment of the dynamic parameters of these complex structures could lead to the disruption of functionality of this costly infrastructure as it happened few years ago [35]. This article presents the evaluation of the modal parameters of the Rayitos de Sol cable-stayed bridge, as a case of study, using the traditional PP and more robust techniques such as the FDD, SSI, RDT, and ITD to compare these techniques side by side and address the current research gap in the local engineering mainstream. This assessment is performed through the development of a three-dimensional finite element model and the analysis of ambient vibration measurements. The recorded signals were analyzed using a set of well-known MIMs. To complement the limited ambient vibration data, the validated finite element model was subjected to a Gaussian white noise to compare the features of the identification methods adopted in this study.
2. DESCRIPTION OF “RAYITOS DE SOL” CABLE-STAYED BRIDGE
The "Rayitos de Sol" cable-stayed bridge is footbridge (Fig. 1) located between the districts of Rímac and Cercado de Lima, in Peru, which has a structure of approximately 216 meters of span.
The structure consists of a cable-stayed span made of a concrete-steel composite deck, an access span which its deck is comprised of prestressed beams and a reinforced concrete slab, reinforced concrete columns supporting the access span, middle and end access reinforced concrete stairs, an access ramp at one end, a reinforced concrete tower that supports the cable-stayed span, and stay cables supporting the main span. In addition, the access span is made up of 5 spans that are joined through expansion joints. More details of the bridge geometry can be found in the work developed by León [36].
3. FINITE ELEMENT MODEL
A three-dimensional finite element model (FEM) was developed based on the geometric and mechanical properties provided by the bridge blueprints and field inspections 36). A view of the constructed model is shown in Fig. 5.
In the modeling of the slabs and beams, shell and beam-column elements were used, respectively. For the exact geometric location of the beams, slabs and columns, offset constraints were used. Fig. 2 shows the beam’s position related to the slab in a typical section of the cable-stayed deck. Likewise, the prestressed beams in the access span were located with respect to the slab. With regard to the columns and tower, beam-column elements were used multiplied by a factor to take into account the cracking of the sections. To assess the effective stiffness of the concrete elements, a moment-curvature analysis was performed using the shape of the cross section, the strain-stress curves of the component materials, the longitudinal and transversal steel layout and the supported axial load [36]. In the case of the columns supporting the access span, the effective stiffness resulted was 34% of the gross section stiffness.
Truss elements were adopted to model stay cables. In this element, an equivalent modulus of elasticity is used to take into account the non-linear behavior of the cables [36]. However, in this case, the reduction in the modulus of elasticity is negligible.
The stairs are monolithically connected to cable-stayed span and supported in reinforced concrete columns. However, the access ramp is simple supported in the access span. In contrast, the expansion joints joining the 5 access spans are connected in a non-monolithic way. These joints establish special boundary conditions, which have been represented through the use of link elements (see Fig. 3) to simulate the relative discontinuity in the deck. More details in the development of the finite element model can be found in León [36].
4. AMBIENT VIBRATION DATA
For the experimental stage, the StrainIndicator measurement equipment was used to record the data on 4 channels (Fig. 4). The equipment's EPROM memory was used to store the data. Later, this data was downloaded through the connection to the Ethernet Network, for which the “StrainIndicator.exe” program was used. Two channels were used which recorded data from speed sensors (Shenck Pickups).
With the development of finite element model and preliminary field visits, strategic measurement locations were chosen. This ensured an adequate identification of the dynamic characteristics. The measurement was made at 11 different points on the structure (Fig. 5), 5 points on the access span and 6 points on the cable-stayed span.
In the field measurements, the pedestrian load was also considered as ambient vibration. A total of 120 measurements were made, of which 40 were used to determine the dynamic characteristics and 80 of maximum accelerations on the access span, all in the vertical and lateral direction (Fig. 6). The data collection frequency entered into the equipment was 100 samples per second.
To complement the limited data recorded, a zero-mean Gaussian white noise was used. Using this artificial excitation, structural responses are simulated and acceleration records are used for modal identification.
5. RESULTS
In the first part of this section, the dynamic parameters of the bridge were assessed using the developed finite element model. Then, the field-recorded data is analyzed through a series of frequency domain (PP, FDD, SSI) and time domain (RDT, ITD) system identification in order to validate the finite element model.
5.1 FINITE ELEMENT MODEL
After the elaboration of the 3D finite element model, the dynamic properties of the bridge are obtained using a modal analysis. In this case, only the first 8 modes of vibration were of interest.
In Fig. 8, the FEM and the first 3 fundamental modal forms are shown. It should be noted that for the precise obtaining of the periods and modes of vibration, the correct designation of the effective stiffnesses and boundary conditions played an important role. This could be verified with the measurements obtained in the field.
5.2 MODAL ANALYSIS USING FIELD-RECORDED DATA
Using the PP only was possible to assess the frequencies as it is shown in Fig. 9 . The modal shapes can be determined by using an arduous analysis of the processed signals and the damping estimation can be performed along with the Half-Power (HP) method [14] [15]. Due to the limited field data recorded, only the damping of the first modes can be considered as reliable values. In comparison with other MIMs, it should be noted that this method forces the user to verify signal by signal the coherence of each obtained results. In the case of the FDD, only frequencies were estimated as no simultaneous measurements were made at different points to estimate the modal shapes and the damping of the first mode was estimated using the RDT. The results of this method are depicted in Fig. 10 and Fig. 11. Likewise, the SSI was not able to capture the modal shapes due to the lack of simultaneous measurements. In the case of the time domain methods, the RDT estimates the damping ratio of the first mode and the ITD was not able to detect any dynamic parameter due to free vibration condition required in the field-vibration data. An attempt was made transforming the random signals using the RDT but the nature and frequency content of the original signal was lost in the process. A summary of results is presented in Table I.
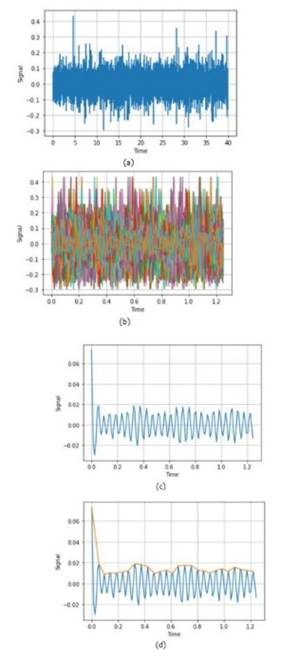
Fig. 11 RDT process. (a) signal. (b) Cut signal. (c) average of signals from (b). (d) Logarithmic decrement approximation. Damping ratio 1.175%
TABLE I Modal parameters comparison
Method | FEM | PP | FDD | SSI | RDT | ITD | ||||||||
---|---|---|---|---|---|---|---|---|---|---|---|---|---|---|
Mode | f | ξ | f | ξ | f | ξ | f | ξ | ξ | f | ξ | |||
1 | 0.836 | 1.15% | 0.830 | 1.300% | 0.7812 | 1.175% | 0.8121 | 31.241% | 1.175% | - | - | |||
2 | 1.455 | 1.15% | 1.563 | 0.883% | 1.172 | 1.175% | 1.7373 | 9.408% | 1.175% | - | - | |||
3 | 1.572 | 1.15% | 1.801 | 0.961% | 1.562 | 1.175% | 2.4338 | 2.022% | 1.175% | - | - | |||
4 | 2.477 | 1.15% | 2.541 | 0.510% | 1.855 | 1.175% | 2.5499 | 0.647% | 1.175% | - | - | |||
5 | 3.033 | 1.15% | 3.175 | 0.541% | 2.148 | 1.175% | 3.1367 | 0.825% | 1.175% | - | - | |||
6 | 3.696 | 1.15% | 3.268 | 0.437% | 3.223 | 1.175% | 4.6387 | 1.556% | 1.175% | - | - | |||
7 | 3.892 | 1.15% | 3.423 | 0.364% | 3.711 | 1.175% | 5.7492 | 4.181% | 1.175% | - | - | |||
8 | 4.372 | 1.15% | 4.218 | 0.390% | 4.199 | 1.175% | 6.1155 | 1.105% | 1.175% | - | - |
Note: f: Frequency (Hz) and ξ: Damping (%)
5.3 MODAL ANALYSIS USING ARTIFICIAL DATA
To complement the ambient vibration data analysis, the validated finite element model of the bridge was subjected to artificial ambient excitations described previously. These simulated vibrations responses were processed with the previous modal identification methods to compare the capabilities of the different MIMs when several measurements are performed at the same time. From the results, it can be noticed that the PP provides an estimation of the frequencies (Fig. 12) and damping ratios using the HP method. In the case of the FDD modal shape forms of the cable-stayed bridge (Fig. 14) was possible due to simultaneous measurements over the deck of the bridge. Similarly, the SSI was able to estimate the frequencies and modal shapes (Fig. 15) due to the available recorded data. In contrast to the experimental data, the ITD in this case was capable of assessing the frequencies and damping ratios of the first six modes of the bridge with reliable accuracy. A summary of the results is presented in Table II.
TABLE II. Comparison of modal parameters of the virtual test
Method | FEM | PP | FDD | SSI | RDT | ITD | |||||||
---|---|---|---|---|---|---|---|---|---|---|---|---|---|
Mode | f | ξ | f | ξ | f | ξ | f | ξ | ξ | f | ξ | ||
1 | 0.836 | 1.15% | 0.835 | 1.585% | 0.830 | 1.176% | 0.849 | 7.369% | 1.176% | 0.8529 | 0.957% | ||
2 | 1.455 | 1.15% | 1.579 | 1.153% | 1.562 | 1.176% | 1.724 | 1.668% | 1.176% | 1.605 | 4.716% | ||
3 | 1.572 | 1.15% | 2.421 | 1.326% | 2.197 | 1.176% | 2.947 | 1.157% | 1.176% | 2.393 | 7.453% | ||
4 | 2.477 | 1.15% | 2.863 | 1.110% | 2.441 | 1.176% | 3.942 | 0.2354% | 1.176% | 2.552 | 1.934% | ||
5 | 3.033 | 1.15% | 3.102 | 1.127% | 2.832 | 1.176% | 4.713 | 1.700% | 1.176% | 3.876 | 1.898% | ||
6 | 3.696 | 1.15% | 3.333 | 1.04% | 3.320 | 1.176% | 6.522 | 1.437% | 1.176% | 5.306 | 2.267% | ||
7 | 3.892 | 1.15% | 3.929 | 0.853% | 3.906 | 1.176% | 10.219 | 1.507% | 1.176% | - | - | ||
8 | 4.372 | 1.15% | 4.5086 | 0.925% | 4.102 | 1.176% | 11.924 | 0.691% | 1.176% | - | - |
Note: f: Frequency (Hz) and ξ: Damping (%)
CONCLUSIONS
In this paper, the Rayitos de Sol cable-stayed bridge was selected as a case study. To evaluate the modal parameters of the bridge, ambient vibration data was gathered from strategic points over the deck. Using the field-recorded data, a series of frequency and time domain system identification methods were carried out and then compared. The collected data was analyzed based on output-only identification methods: peak-picking (PP), frequency domain decomposition (FDD), stochastic subspace identification (SSI), random decrement (RD), and Ibrahim time domain identification (ITD). To complement the ambient vibration data analysis, the validated finite element model of the bridge was subjected to artificial ambient excitations to make a comprehensive comparison of the identification methods. The main conclusion of this research may be summarized as follow:
- From the obtained results, the numerical techniques show good agreement with the experimental measurements in the assessment of the frequencies. Moreover, the measured low damping values of the first modes range from 1% to 2% as expected for this type of structure with steel and prestressed concrete elements.
- In the development of the numerical model, considering the effective stiffness of the concrete reinforced elements as well as modelling the right boundary conditions at the expansion joints were the main factors to achieve a good correlation with the measured modal parameters.
- It can be seen that the environmental measurements and the numerical models are capable of capturing the occurrence of very close modal frequencies indicating the existence of modal coupling. This dynamic characteristic has been observed in various cable-stayed bridges.
- Prior to the use of any method, the sensitivity of the data to be considered in its use must be known. It has been seen that parameters such as the number of modes, number of signals, number of columns and rows in the internal matrix arrangements, sampling frequency, etc. have an impact on the results obtained. Simulated analyzes such as the one performed in this investigation will give a general overview of the correct number of parameters to consider prior to the fieldwork.
- Similarly, the number of sensors in simultaneous measurement points plays a fundamental role in obtaining precise modal parameters, especially in the modal shapes of the structures. In this sense, developing a FE Model prior to the fieldwork will help in assessing the correct locations of the measurement points in the structure. This would improve the identification of modal parameters.